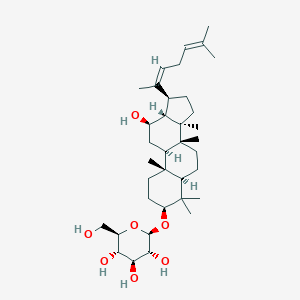
Ginsenoside Rh3
Overview
Description
Ginsenoside Rh3 is a rare ginsenoside derived from the Panax ginseng plant, which belongs to the Araliaceae family. Ginsenosides are triterpenoid saponins that are known for their diverse pharmacological properties. This compound, in particular, has attracted significant attention due to its potential therapeutic effects, including anti-inflammatory, anti-tumor, and anti-metastatic properties .
Mechanism of Action
Target of Action
Ginsenoside Rh3, a rare ginsenoside extracted from Panax notoginseng, has been found to target the Extracellular Signal-Regulated Kinase (ERK) . ERK is a key protein in the MAPK/ERK pathway, which plays a crucial role in regulating cell functions such as proliferation, differentiation, and survival .
Mode of Action
This compound interacts with its target, ERK, to inhibit lung cancer metastasis . It has also been found to enhance the sensitivity of hepatocellular carcinoma cells to regorafenib-induced anticancer effects by promoting apoptosis . This suggests that this compound can modulate the activity of ERK, thereby influencing cell proliferation and metastasis .
Biochemical Pathways
The major biochemical pathways affected by this compound involve the ERK pathway and the JAK/STAT3 signaling pathway . By targeting ERK, this compound can inhibit lung cancer metastasis . Additionally, it has been found to inhibit the JAK/STAT3 signaling pathway, thereby increasing the susceptibility of cancer cells to regorafenib-induced death .
Pharmacokinetics
They are also quickly cleared from the body . These properties may impact the bioavailability and efficacy of this compound.
Result of Action
The primary result of this compound’s action is the inhibition of cancer cell proliferation and metastasis . It has been found to significantly inhibit lung cancer metastasis both in vivo and in vitro . Additionally, it enhances the sensitivity of hepatocellular carcinoma cells to regorafenib-induced anticancer effects by promoting apoptosis .
Action Environment
The action of this compound can be influenced by various environmental factors. For instance, the compound’s stability and efficacy can be affected by temperature . Furthermore, the compound’s action can also be influenced by the presence of other compounds, as seen in its enhanced effect when combined with regorafenib in treating hepatocellular carcinoma .
Biochemical Analysis
Biochemical Properties
Ginsenoside Rh3 interacts with various enzymes, proteins, and other biomolecules. For instance, it has been found to regulate ROS-mediated PI3K/AKT/mTOR, which is involved in the apoptosis pathway . This interaction is associated with the downregulation of Bcl-2 and upregulation of Caspase-3, Caspase-7, Cleaved PARP, and Bax in tumor cells .
Cellular Effects
This compound has significant effects on various types of cells and cellular processes. It has been found to inhibit HCT116 cell proliferation, invasion, and migration, arrest cells at G1 phase, and increase apoptosis . It also has the potential to reverse insulin resistance .
Molecular Mechanism
At the molecular level, this compound exerts its effects through several mechanisms. It regulates ROS-mediated PI3K/AKT/mTOR, which is involved in the apoptosis pathway . This regulation is associated with the downregulation of Bcl-2 and upregulation of Caspase-3, Caspase-7, Cleaved PARP, and Bax in tumor cells .
Temporal Effects in Laboratory Settings
In laboratory settings, this compound has shown changes in its effects over time. For instance, it has been found to enhance the sensitivity of HCC cells to regorafenib-induced anticancer effects by promoting apoptosis .
Dosage Effects in Animal Models
In animal models, the effects of this compound vary with different dosages. For example, it has been found that the concentration of Rh3 above 50 μM exerted a significant effect on the proliferation capacity of A549 and PC9 cells .
Metabolic Pathways
This compound is involved in various metabolic pathways. It has been found to be involved in the biosynthesis of rare ginsenosides . The key enzymes involved in the biosynthetic pathways of rare ginsenosides have been identified, and these include glycosyltransferases .
Preparation Methods
Synthetic Routes and Reaction Conditions: Ginsenoside Rh3 can be synthesized through the enzymatic transformation of other ginsenosides. Enzymes such as β-glucosidase, β-xylosidase, α-l-arabinofuranosidase, and α-l-rhamnosidase are used to hydrolyze the side chain glycogroups of ginsenosides . Additionally, acid transformation methods can be employed, where specific conditions such as temperature, time, and acid concentration are optimized to produce this compound .
Industrial Production Methods: The industrial production of this compound involves the use of microbial cultures and plant extraction techniques. The glycosidases from microbial cultures are particularly effective in hydrolyzing the side chains of ginsenosides to produce this compound. This method is advantageous for large-scale production due to its efficiency and cost-effectiveness .
Chemical Reactions Analysis
Types of Reactions: Ginsenoside Rh3 undergoes various chemical reactions, including oxidation, reduction, and substitution. These reactions are essential for modifying the compound’s structure and enhancing its pharmacological properties.
Common Reagents and Conditions:
Oxidation: Common oxidizing agents such as hydrogen peroxide and potassium permanganate are used under controlled conditions to oxidize this compound.
Reduction: Reducing agents like sodium borohydride and lithium aluminum hydride are employed to reduce specific functional groups in this compound.
Substitution: Substitution reactions often involve nucleophiles such as hydroxide ions or amines under basic conditions.
Major Products Formed: The major products formed from these reactions include various derivatives of this compound, which may exhibit enhanced or altered pharmacological activities .
Scientific Research Applications
Ginsenoside Rh3 has a wide range of scientific research applications across various fields:
Chemistry:
- Used as a precursor for synthesizing other bioactive compounds.
- Studied for its unique chemical properties and potential for chemical modifications.
Biology:
- Investigated for its role in cellular signaling pathways and gene expression.
- Used in studies related to cell proliferation, apoptosis, and differentiation.
Medicine:
- Exhibits anti-inflammatory and anti-tumor effects, making it a potential candidate for cancer therapy .
- Studied for its ability to inhibit metastasis in lung cancer cells by targeting extracellular signal-regulated kinase .
Industry:
- Used in the development of health supplements and functional foods.
- Explored for its potential in cosmetic formulations due to its anti-aging properties.
Comparison with Similar Compounds
Ginsenoside Rh3 is unique among ginsenosides due to its specific structure and pharmacological properties. Similar compounds include:
Ginsenoside Rg3: Known for its anti-tumor and anti-metastatic effects.
Ginsenoside Rh2: Exhibits strong anti-cancer properties and is used in cancer therapy.
Ginsenoside Rg5: Studied for its anti-inflammatory and anti-oxidative effects.
Compared to these compounds, this compound has shown a higher efficacy in inhibiting lung cancer metastasis and modulating specific signaling pathways .
Properties
IUPAC Name |
(2R,3S,4S,5R,6R)-2-(hydroxymethyl)-6-[[(3S,5R,8R,9R,10R,12R,13R,14R,17S)-12-hydroxy-4,4,8,10,14-pentamethyl-17-[(2Z)-6-methylhepta-2,5-dien-2-yl]-2,3,5,6,7,9,11,12,13,15,16,17-dodecahydro-1H-cyclopenta[a]phenanthren-3-yl]oxy]oxane-3,4,5-triol | |
---|---|---|
Source | PubChem | |
URL | https://pubchem.ncbi.nlm.nih.gov | |
Description | Data deposited in or computed by PubChem | |
InChI |
InChI=1S/C36H60O7/c1-20(2)10-9-11-21(3)22-12-16-36(8)28(22)23(38)18-26-34(6)15-14-27(33(4,5)25(34)13-17-35(26,36)7)43-32-31(41)30(40)29(39)24(19-37)42-32/h10-11,22-32,37-41H,9,12-19H2,1-8H3/b21-11-/t22-,23-,24-,25+,26-,27+,28+,29-,30+,31-,32+,34+,35-,36-/m1/s1 | |
Source | PubChem | |
URL | https://pubchem.ncbi.nlm.nih.gov | |
Description | Data deposited in or computed by PubChem | |
InChI Key |
PHLXREOMFNVWOH-YAGNRYSRSA-N | |
Source | PubChem | |
URL | https://pubchem.ncbi.nlm.nih.gov | |
Description | Data deposited in or computed by PubChem | |
Canonical SMILES |
CC(=CCC=C(C)C1CCC2(C1C(CC3C2(CCC4C3(CCC(C4(C)C)OC5C(C(C(C(O5)CO)O)O)O)C)C)O)C)C | |
Source | PubChem | |
URL | https://pubchem.ncbi.nlm.nih.gov | |
Description | Data deposited in or computed by PubChem | |
Isomeric SMILES |
CC(=CC/C=C(/C)\[C@H]1CC[C@@]2([C@@H]1[C@@H](C[C@H]3[C@]2(CC[C@@H]4[C@@]3(CC[C@@H](C4(C)C)O[C@H]5[C@@H]([C@H]([C@@H]([C@H](O5)CO)O)O)O)C)C)O)C)C | |
Source | PubChem | |
URL | https://pubchem.ncbi.nlm.nih.gov | |
Description | Data deposited in or computed by PubChem | |
Molecular Formula |
C36H60O7 | |
Source | PubChem | |
URL | https://pubchem.ncbi.nlm.nih.gov | |
Description | Data deposited in or computed by PubChem | |
DSSTOX Substance ID |
DTXSID401317008 | |
Record name | Ginsenoside Rh3 | |
Source | EPA DSSTox | |
URL | https://comptox.epa.gov/dashboard/DTXSID401317008 | |
Description | DSSTox provides a high quality public chemistry resource for supporting improved predictive toxicology. | |
Molecular Weight |
604.9 g/mol | |
Source | PubChem | |
URL | https://pubchem.ncbi.nlm.nih.gov | |
Description | Data deposited in or computed by PubChem | |
CAS No. |
105558-26-7 | |
Record name | Ginsenoside Rh3 | |
Source | CAS Common Chemistry | |
URL | https://commonchemistry.cas.org/detail?cas_rn=105558-26-7 | |
Description | CAS Common Chemistry is an open community resource for accessing chemical information. Nearly 500,000 chemical substances from CAS REGISTRY cover areas of community interest, including common and frequently regulated chemicals, and those relevant to high school and undergraduate chemistry classes. This chemical information, curated by our expert scientists, is provided in alignment with our mission as a division of the American Chemical Society. | |
Explanation | The data from CAS Common Chemistry is provided under a CC-BY-NC 4.0 license, unless otherwise stated. | |
Record name | Ginsenoside Rh3 | |
Source | ChemIDplus | |
URL | https://pubchem.ncbi.nlm.nih.gov/substance/?source=chemidplus&sourceid=0105558267 | |
Description | ChemIDplus is a free, web search system that provides access to the structure and nomenclature authority files used for the identification of chemical substances cited in National Library of Medicine (NLM) databases, including the TOXNET system. | |
Record name | Ginsenoside Rh3 | |
Source | EPA DSSTox | |
URL | https://comptox.epa.gov/dashboard/DTXSID401317008 | |
Description | DSSTox provides a high quality public chemistry resource for supporting improved predictive toxicology. | |
Retrosynthesis Analysis
AI-Powered Synthesis Planning: Our tool employs the Template_relevance Pistachio, Template_relevance Bkms_metabolic, Template_relevance Pistachio_ringbreaker, Template_relevance Reaxys, Template_relevance Reaxys_biocatalysis model, leveraging a vast database of chemical reactions to predict feasible synthetic routes.
One-Step Synthesis Focus: Specifically designed for one-step synthesis, it provides concise and direct routes for your target compounds, streamlining the synthesis process.
Accurate Predictions: Utilizing the extensive PISTACHIO, BKMS_METABOLIC, PISTACHIO_RINGBREAKER, REAXYS, REAXYS_BIOCATALYSIS database, our tool offers high-accuracy predictions, reflecting the latest in chemical research and data.
Strategy Settings
Precursor scoring | Relevance Heuristic |
---|---|
Min. plausibility | 0.01 |
Model | Template_relevance |
Template Set | Pistachio/Bkms_metabolic/Pistachio_ringbreaker/Reaxys/Reaxys_biocatalysis |
Top-N result to add to graph | 6 |
Feasible Synthetic Routes
Disclaimer and Information on In-Vitro Research Products
Please be aware that all articles and product information presented on BenchChem are intended solely for informational purposes. The products available for purchase on BenchChem are specifically designed for in-vitro studies, which are conducted outside of living organisms. In-vitro studies, derived from the Latin term "in glass," involve experiments performed in controlled laboratory settings using cells or tissues. It is important to note that these products are not categorized as medicines or drugs, and they have not received approval from the FDA for the prevention, treatment, or cure of any medical condition, ailment, or disease. We must emphasize that any form of bodily introduction of these products into humans or animals is strictly prohibited by law. It is essential to adhere to these guidelines to ensure compliance with legal and ethical standards in research and experimentation.