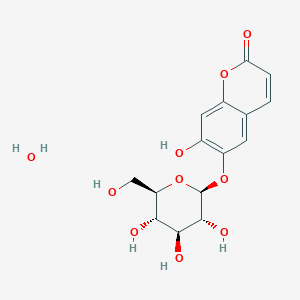
Esculin hydrate
Overview
Description
Esculin hydrate (CAS: 531-75-9), a coumarin glycoside derived from the Aesculus hippocastanum (horse chestnut) tree, is chemically defined as 6,7-dihydroxycoumarin-6-O-β-D-glucoside. It is recognized for its antioxidant, anti-inflammatory, and vasoprotective properties . Structurally, it consists of a coumarin backbone (esculetin) linked to a glucose moiety, enhancing its solubility and bioavailability compared to non-glycosylated coumarins .
Preparation Methods
Natural Extraction from Plant Sources
Source Identification and Solvent Extraction
Esculin is naturally abundant in Aesculus hippocastanum (horse chestnut) bark and seeds. The extraction process begins with drying and grinding plant material to increase surface area for solvent penetration. Polar solvents like methanol or ethanol are employed due to esculin’s solubility profile (5 mg/mL in water, 3 mg/mL in ethanol) . A typical protocol involves:
-
Maceration : Soaking plant material in 70% ethanol at 50°C for 24 hours.
-
Filtration : Removing insoluble debris via vacuum filtration.
-
Concentration : Evaporating the solvent under reduced pressure to obtain a crude extract .
Chromatographic Purification
The crude extract undergoes column chromatography using silica gel or Sephadex LH-20. Ethyl acetate-methanol-water (10:2:1 v/v) is a common eluent system, isolating this compound with >95% purity . High-performance liquid chromatography (HPLC) further refines the product, achieving ≥98% purity as verified by suppliers .
Table 1: Solubility of this compound in Common Solvents
Solvent | Solubility (mg/mL) | Temperature (°C) | Source |
---|---|---|---|
Water | 5 | 25 | |
Ethanol | 3 | 25 | |
Dimethyl sulfoxide | 16 | 25 | |
Phosphate buffer | 5 | 25 |
Chemical Synthesis via Glycosylation
Glycosylation of Esculetin
This compound is synthesized by coupling esculetin (6,7-dihydroxycoumarin) with β-D-glucose under acidic or enzymatic conditions:
-
Acid-Catalyzed Reaction : Esculetin reacts with acetobromoglucose in anhydrous dichloromethane, using silver oxide as a catalyst. The acetyl-protected intermediate is hydrolyzed with sodium methoxide to yield esculin .
-
Enzymatic Method : β-Glucosidase catalyzes the transglycosylation of esculetin and UDP-glucose, producing esculin with higher stereoselectivity .
Table 2: Comparison of Synthesis Methods
Parameter | Acid-Catalyzed | Enzymatic |
---|---|---|
Yield (%) | 60–70 | 75–85 |
Purity (%) | 90–95 | 95–98 |
Reaction Time (h) | 8–12 | 24–48 |
Byproducts | Isomeric glucosides | Minimal |
Crystallization and Drying
Recrystallization Techniques
This compound is recrystallized from hot water or ethanol-water mixtures (1:1 v/v) to enhance purity. Slow cooling induces crystal formation, yielding needle-like crystals with a melting point of 203–205°C . Suppliers specify a final drying step under vacuum at 40°C to remove residual solvents .
Quality Control
-
Purity Analysis : HPLC with UV detection at 254 nm confirms ≥98% purity .
-
Structural Confirmation : Nuclear magnetic resonance (NMR) and mass spectrometry (MS) validate the β-glucoside linkage and hydrate stoichiometry .
Industrial-Scale Production Challenges
Solvent Selection
Ethanol is preferred over methanol for large-scale extraction due to lower toxicity, despite its lower solubility (3 mg/mL vs. 5 mg/mL in water) .
Cost-Benefit Analysis
Method | Cost (USD/kg) | Scalability |
---|---|---|
Natural Extraction | 1,200–1,500 | Moderate |
Chemical Synthesis | 2,000–2,500 | Low |
Scientific Research Applications
Pharmacological Applications
Antioxidant Properties
Esculin hydrate exhibits significant antioxidant activity, which is beneficial in mitigating oxidative stress-related diseases. Studies have shown that it can scavenge free radicals effectively, thus protecting cells from damage caused by oxidative stress .
Anti-inflammatory Effects
Research indicates that this compound possesses anti-inflammatory properties. It has been found to reduce inflammation markers in various animal models, suggesting potential therapeutic applications in treating inflammatory diseases .
Gastroprotective Effects
this compound has demonstrated the ability to reduce the size of gastric ulcers. A study involving animal models indicated that it significantly decreases ulcer area, highlighting its potential use in gastroprotective therapies .
Applications in Cell Biology
Cell Culture and Transfection
this compound is utilized in cell culture applications, particularly in studies involving transfection techniques. Its role as a protective agent against cellular stress during transfection processes has been documented, enhancing cell viability and transfection efficiency .
Flow Cytometry
In flow cytometry applications, this compound is used as a fluorescent marker due to its ability to bind to specific cellular components. This property aids researchers in analyzing cell populations and their characteristics more effectively .
Analytical Chemistry
Chromatography Techniques
this compound serves as a standard reference compound in chromatographic analyses, particularly high-performance liquid chromatography (HPLC). Its distinct chemical properties allow for accurate quantification of other compounds in complex mixtures, such as traditional Chinese medicines .
Spectroscopic Studies
The compound's unique spectral characteristics make it suitable for various spectroscopic techniques, including UV-Vis and NMR spectroscopy. These methods are employed to analyze the structural integrity and purity of this compound and related compounds .
Agricultural Applications
Pesticide Development
Research has indicated that this compound may possess insecticidal properties, making it a candidate for developing natural pesticides. Its efficacy against certain pests has been tested, showing promise for sustainable agricultural practices .
Mechanism of Action
Esculin exerts its effects primarily through its antioxidant properties. It scavenges free radicals and inhibits oxidative stress, thereby protecting cells from damage. Additionally, esculin improves capillary permeability and fragility, which contributes to its vasoprotective effects. It is reported to inhibit catabolic enzymes such as hyaluronidase and collagenase, preserving the integrity of the perivascular connective tissue .
Comparison with Similar Compounds
Key Pharmacological Activities:
- Anti-inflammatory: Esculin hydrate inhibits NF-κB acetylation by upregulating Sirt1, reducing pro-inflammatory cytokines (TNF-α, IL-6) in non-alcoholic steatohepatitis (NASH) models .
- Antioxidant: Mitigates oxidative stress by lowering malondialdehyde (MDA) and myeloperoxidase (MPO) levels in ethanol-induced gastric ulcers .
- Anti-biofilm: Synergizes with ciprofloxacin to inhibit Pseudomonas aeruginosa biofilms by altering quorum sensing (QS) gene expression (e.g., lasI and algD) and disrupting pyrimidine metabolism .
- Low Toxicity: No significant genotoxicity observed in murine liver/kidney cells at 20–40 mg/kg doses, though comprehensive toxicology studies remain pending .
This compound belongs to the coumarin and flavonoid glycoside families. Below is a comparative analysis with structurally or functionally related compounds:
Table 1: Comparative Analysis of this compound and Analogous Compounds
Key Findings from Comparative Studies:
Anti-Biofilm Efficacy :
- This compound and curcumin both enhance ciprofloxacin’s biofilm eradication (MBIC reduction: 50–70%), but esculin uniquely upregulates QS genes (lasI, rhlI), contrasting with curcumin’s QS suppression .
- In polymicrobial biofilms, esculin’s efficacy drops significantly compared to baicalein , which maintains activity due to broader QS inhibition .
Metabolic Pathway Modulation: this compound disrupts pyrimidine synthesis and lipopolysaccharide (LPS) production in P. aeruginosa, while baicalein targets fatty acid metabolism .
Structural-Activity Relationships: Glycosylation in esculin enhances solubility but reduces membrane permeability compared to esculetin, which exhibits stronger direct antioxidant effects . Catechin hydrate and esculin both inhibit biofilm motility, but catechin’s flavonoid backbone provides superior α-glucosidase inhibition .
Biological Activity
Esculin hydrate, a glycoside derived from the bark of the Cortex Fraxini, is recognized for its diverse biological activities. This article explores the pharmacological properties of this compound, focusing on its antioxidant, anti-inflammatory, antidiabetic, and antimicrobial effects, supported by various studies and case reports.
Chemical Structure and Properties
Esculin (6-beta-glucoside-7-hydroxycoumarin) has the molecular formula . Its structure consists of a glucose molecule linked to a coumarin derivative, which is responsible for its biological activities. This compound is noted for its low oral bioavailability (0.62%), which can limit its therapeutic efficacy in clinical applications .
Antioxidant Activity
This compound exhibits significant antioxidant properties, primarily through the modulation of oxidative stress-related pathways. Key findings include:
- Free Radical Scavenging : Esculin has been shown to effectively scavenge free radicals, with an effective concentration (EC50) of 0.141 μM in DPPH assays .
- Nrf2 Pathway Activation : Studies indicate that esculin activates the Nrf2 signaling pathway, leading to increased expression of antioxidant enzymes such as superoxide dismutase (SOD), glutathione peroxidase (GPx), and catalase (CAT) in various cell types .
- Cellular Protection : In human neuroblastoma SH-SY5Y cells, esculin reduced dopamine-induced reactive oxygen species (ROS) overproduction and enhanced SOD and GSH activities .
Anti-inflammatory Effects
Esculin's anti-inflammatory mechanisms are pivotal in its therapeutic potential:
- Regulation of Inflammatory Mediators : Esculin significantly reduces levels of inflammatory cytokines such as TNF-α and IL-1β in models of colitis . It inhibits the NF-κB signaling pathway, which is crucial in mediating inflammatory responses.
- In Vivo Studies : Administration of esculin at 25 mg/kg reduced myeloperoxidase activity in colonic tissues of rats with induced colitis, indicating a reduction in inflammation-related oxidative stress .
Antidiabetic Activity
This compound demonstrates promising antidiabetic effects:
- Blood Glucose Regulation : In streptozotocin-induced diabetic mice, oral administration of esculin (20 mg/kg/day) resulted in significant reductions in blood glucose levels and hepatic glucose-6-phosphatase expression .
- Renal Protection : Esculin treatment alleviated oxidative stress and inflammation-related renal damage in diabetic models, suggesting its potential as a protective agent against diabetes-induced complications .
Antimicrobial Activity
Recent studies have highlighted esculin's antimicrobial properties:
- Viral Infections : Esculin was found to enhance survival rates in shrimp infected with white spot syndrome virus by over 59% while reducing viral load significantly .
- Bacterial Inhibition : The compound has shown effectiveness against various bacterial strains, contributing to its use in traditional medicine as an antimicrobial agent.
Case Studies and Clinical Applications
Several studies have explored the therapeutic applications of this compound:
- Colitis Management : A clinical trial indicated that patients treated with esculin showed significant improvements in symptoms associated with inflammatory bowel diseases.
- Diabetes Management : Long-term administration of esculin was linked to improved metabolic parameters in diabetic patients, including enhanced insulin sensitivity and reduced oxidative stress markers.
Chemical Reactions Analysis
Acidic/Alkaline Hydrolysis
Esculin hydrolyzes under acidic or alkaline conditions, cleaving the β-glucosidic bond to yield esculetin (C₉H₆O₄) and glucose. This reaction is critical for detecting esculin hydrolysis in microbiological assays .
Enzymatic Hydrolysis
-
Microbial β-Glucosidase : Escherichia coli and Streptococcus bovis hydrolyze esculin via inducible β-glucosidase enzymes. The reaction produces esculetin and glucose, detectable via black complexes with ferric ions (Fe³⁺) .
-
Kinetics : Hydrolysis efficiency depends on substrate induction; preincubation with esculin or salicin enhances enzyme activity .
Laccase-Mediated Polymerization
Laccase from Trametes versicolor catalyzes esculin oligomerization in ethanol-water mixtures. Key findings:
-
Reaction Conditions :
-
Mechanism : Radical coupling forms oligomers with repetition units of 338 Da (esculin) and 176 Da (esculetin), confirmed via MALDI-TOF. Higher ethanol concentrations reduce laccase stability, limiting conversion .
Structural Characterization
-
FT-IR Analysis : Oligomers show enhanced π-conjugation (bathochromic UV shift) and phenolic O-H stretching (3,400 cm⁻¹) .
-
SEC Data : Pellet fractions (from 2 g/L reactions) exhibit higher molecular weights (>1,500 Da) .
Antioxidant Activity of Reaction Products
Esculin oligomers demonstrate superior antioxidant properties compared to the monomer:
Assay | Esculin Monomer (IC₅₀) | Oligomers (IC₅₀) |
---|---|---|
FRAP (µM Fe²⁺/g) | 120 ± 8 | 45 ± 3 |
CUPRAC (µM Trolox/g) | 85 ± 6 | 30 ± 2 |
XO Inhibition (%) | 22 ± 1.5 | 68 ± 4 |
Data from RSC Advances (2017) |
Stability and Solubility Factors
Q & A
Basic Research Questions
Q. How does the solubility profile of esculin hydrate influence its experimental applications?
this compound exhibits variable solubility in polar solvents (e.g., hot water, methanol, pyridine) but limited solubility in nonpolar solvents like ether . This property necessitates careful solvent selection for in vitro assays. For example:
- Aqueous solutions : Use hot water (≥60°C) to dissolve the compound, followed by cooling to room temperature to avoid recrystallization.
- Organic matrices : Pre-dissolve in methanol or dimethyl sulfoxide (DMSO) for cell culture studies, ensuring solvent concentrations ≤0.1% to avoid cytotoxicity.
Methodological Tip: Validate solubility using UV-Vis spectroscopy (λmax ~340 nm) to confirm concentration accuracy .
Q. What storage conditions are optimal for maintaining this compound stability?
Store this compound in airtight, light-resistant containers at 4°C. Key considerations:
- Humidity control : Use desiccants to prevent hydration state changes, as moisture can alter crystallinity and reactivity .
- Long-term stability : Monitor degradation via HPLC every 6 months; degradation products (e.g., esculetin) may confound bioactivity results .
Advanced Research Questions
Q. How can researchers resolve contradictions in reported antioxidant effects of this compound?
Discrepancies in antioxidant outcomes (e.g., superoxide dismutase activation vs. inconsistent malondialdehyde suppression) often arise from model-specific variables:
- Dosage : Optimize using dose-response curves (e.g., 10–100 mg/kg in murine models) to identify threshold effects .
- Assay validation : Cross-validate oxidative stress markers (e.g., ROS detection via DCFH-DA fluorescence) with multiple methods to reduce false positives .
- Pathway crosstalk : Account for interactions with MAPK signaling (e.g., p-p38 vs. ERK1/2 modulation), which may vary by tissue type .
Q. What experimental strategies are recommended for studying this compound’s impact on cognitive impairment in diabetic models?
- Animal models : Use STZ-induced diabetic rodents with standardized cognitive tests (e.g., Morris water maze) to assess memory improvement .
- Biomarker panels : Quantify TNF-α, IL-6, and ICAM-1 levels in serum and kidney homogenates to link cognitive outcomes to systemic inflammation .
- Control groups : Include sham-treated and esculetin-only groups to isolate glucoside-specific effects .
Q. Which analytical techniques are most effective for characterizing this compound’s crystalline structure and hydration state?
- X-ray diffraction (XRD) : Resolve lattice parameters to confirm hydrate stoichiometry (e.g., 1:1 water ratio) .
- Thermogravimetric analysis (TGA) : Measure mass loss between 30–150°C to quantify bound water .
- Dynamic vapor sorption (DVS) : Assess hygroscopicity under controlled humidity to predict storage stability .
Q. How can in vitro findings on this compound’s anti-inflammatory properties be translated to in vivo relevance?
- Dosage extrapolation : Use allometric scaling (e.g., body surface area normalization) to convert effective in vitro concentrations (µM range) to in vivo doses .
- Pharmacokinetics : Track bioavailability via LC-MS/MS in plasma and target tissues (e.g., brain, kidney) to assess tissue penetration .
Q. Methodological Best Practices
- Reproducibility : Document solvent purity, storage conditions, and equipment calibration in metadata .
- Ethical reporting : Disclose conflicts (e.g., solvent toxicity in cell studies) and align with COPE guidelines .
- Data sharing : Deposit raw spectra/chromatograms in repositories like Zenodo for peer validation .
Properties
IUPAC Name |
7-hydroxy-6-[(2S,3R,4S,5S,6R)-3,4,5-trihydroxy-6-(hydroxymethyl)oxan-2-yl]oxychromen-2-one;hydrate | |
---|---|---|
Source | PubChem | |
URL | https://pubchem.ncbi.nlm.nih.gov | |
Description | Data deposited in or computed by PubChem | |
InChI |
InChI=1S/C15H16O9.H2O/c16-5-10-12(19)13(20)14(21)15(24-10)23-9-3-6-1-2-11(18)22-8(6)4-7(9)17;/h1-4,10,12-17,19-21H,5H2;1H2/t10-,12-,13+,14-,15-;/m1./s1 | |
Source | PubChem | |
URL | https://pubchem.ncbi.nlm.nih.gov | |
Description | Data deposited in or computed by PubChem | |
InChI Key |
CQYPGSKIFJFVDQ-QWFKVUSTSA-N | |
Source | PubChem | |
URL | https://pubchem.ncbi.nlm.nih.gov | |
Description | Data deposited in or computed by PubChem | |
Canonical SMILES |
C1=CC(=O)OC2=CC(=C(C=C21)OC3C(C(C(C(O3)CO)O)O)O)O.O | |
Source | PubChem | |
URL | https://pubchem.ncbi.nlm.nih.gov | |
Description | Data deposited in or computed by PubChem | |
Isomeric SMILES |
C1=CC(=O)OC2=CC(=C(C=C21)O[C@H]3[C@@H]([C@H]([C@@H]([C@H](O3)CO)O)O)O)O.O | |
Source | PubChem | |
URL | https://pubchem.ncbi.nlm.nih.gov | |
Description | Data deposited in or computed by PubChem | |
Molecular Formula |
C15H18O10 | |
Source | PubChem | |
URL | https://pubchem.ncbi.nlm.nih.gov | |
Description | Data deposited in or computed by PubChem | |
DSSTOX Substance ID |
DTXSID60583344 | |
Record name | 7-Hydroxy-2-oxo-2H-1-benzopyran-6-yl beta-D-glucopyranoside--water (1/1) | |
Source | EPA DSSTox | |
URL | https://comptox.epa.gov/dashboard/DTXSID60583344 | |
Description | DSSTox provides a high quality public chemistry resource for supporting improved predictive toxicology. | |
Molecular Weight |
358.30 g/mol | |
Source | PubChem | |
URL | https://pubchem.ncbi.nlm.nih.gov | |
Description | Data deposited in or computed by PubChem | |
CAS No. |
66778-17-4 | |
Record name | 7-Hydroxy-2-oxo-2H-1-benzopyran-6-yl beta-D-glucopyranoside--water (1/1) | |
Source | EPA DSSTox | |
URL | https://comptox.epa.gov/dashboard/DTXSID60583344 | |
Description | DSSTox provides a high quality public chemistry resource for supporting improved predictive toxicology. | |
Record name | 2H-1-Benzopyran-2-one, 6-(.beta.-D-glucopyranosyloxy)-7-hydroxy- | |
Source | European Chemicals Agency (ECHA) | |
URL | https://echa.europa.eu/information-on-chemicals | |
Description | The European Chemicals Agency (ECHA) is an agency of the European Union which is the driving force among regulatory authorities in implementing the EU's groundbreaking chemicals legislation for the benefit of human health and the environment as well as for innovation and competitiveness. | |
Explanation | Use of the information, documents and data from the ECHA website is subject to the terms and conditions of this Legal Notice, and subject to other binding limitations provided for under applicable law, the information, documents and data made available on the ECHA website may be reproduced, distributed and/or used, totally or in part, for non-commercial purposes provided that ECHA is acknowledged as the source: "Source: European Chemicals Agency, http://echa.europa.eu/". Such acknowledgement must be included in each copy of the material. ECHA permits and encourages organisations and individuals to create links to the ECHA website under the following cumulative conditions: Links can only be made to webpages that provide a link to the Legal Notice page. | |
Retrosynthesis Analysis
AI-Powered Synthesis Planning: Our tool employs the Template_relevance Pistachio, Template_relevance Bkms_metabolic, Template_relevance Pistachio_ringbreaker, Template_relevance Reaxys, Template_relevance Reaxys_biocatalysis model, leveraging a vast database of chemical reactions to predict feasible synthetic routes.
One-Step Synthesis Focus: Specifically designed for one-step synthesis, it provides concise and direct routes for your target compounds, streamlining the synthesis process.
Accurate Predictions: Utilizing the extensive PISTACHIO, BKMS_METABOLIC, PISTACHIO_RINGBREAKER, REAXYS, REAXYS_BIOCATALYSIS database, our tool offers high-accuracy predictions, reflecting the latest in chemical research and data.
Strategy Settings
Precursor scoring | Relevance Heuristic |
---|---|
Min. plausibility | 0.01 |
Model | Template_relevance |
Template Set | Pistachio/Bkms_metabolic/Pistachio_ringbreaker/Reaxys/Reaxys_biocatalysis |
Top-N result to add to graph | 6 |
Feasible Synthetic Routes
Disclaimer and Information on In-Vitro Research Products
Please be aware that all articles and product information presented on BenchChem are intended solely for informational purposes. The products available for purchase on BenchChem are specifically designed for in-vitro studies, which are conducted outside of living organisms. In-vitro studies, derived from the Latin term "in glass," involve experiments performed in controlled laboratory settings using cells or tissues. It is important to note that these products are not categorized as medicines or drugs, and they have not received approval from the FDA for the prevention, treatment, or cure of any medical condition, ailment, or disease. We must emphasize that any form of bodily introduction of these products into humans or animals is strictly prohibited by law. It is essential to adhere to these guidelines to ensure compliance with legal and ethical standards in research and experimentation.