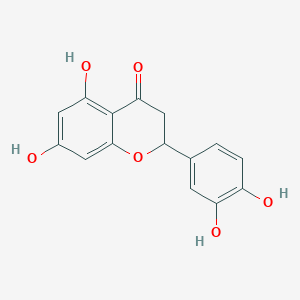
Eriodictyol
Overview
Description
Eriodictyol is a naturally occurring flavonoid, a type of polyphenol with antioxidant and anti-inflammatory properties. It is found in a variety of plants, including citrus fruits and herbs such as thyme, oregano, and rosemary. This compound has been studied for its potential health benefits, including its ability to reduce inflammation, protect against oxidative stress, and improve cognitive function.
Scientific Research Applications
Protection Against Oxidative Stress in Human Cells : Eriodictyol induces long-term protection in ARPE-19 cells against oxidative stress by activating Nrf2 and enhancing the expression of phase 2 proteins, such as heme-oxygenase (HO)-1 and NAD(P)H:quinone oxidoreductase (NQO)-1 (Johnson, Maher, & Hanneken, 2009).
Inhibition of Glioblastoma Cell Migration and Invasion : It suppresses glioblastoma migration and invasion by reversing epithelial-mesenchymal transition (EMT) and downregulating the P38 MAPK/GSK-3β/ZEB1 pathway (Lv et al., 2021).
Modulation of miRNA and mRNA in Human Endothelial Cells : this compound modulates miRNA and mRNA expression, providing insights into its molecular mechanisms of action and potential use in vascular disease therapy (Lee et al., 2017).
Potential Antidiabetic Properties : It shows potential in treating type 2 diabetes by increasing insulin-stimulated glucose uptake and improving insulin resistance in human hepatocellular liver carcinoma cells and differentiated 3T3-L1 adipocytes (Zhang et al., 2012).
Amelioration of Arthritis Symptoms : this compound significantly reduces joint swelling and suppresses the release of inflammatory cytokines in a rat model of collagen-induced arthritis (Lei et al., 2020).
Protection Against Oxidative Stress-Induced Endothelial Injury : It protects endothelial cells against oxidative stress by upregulating heme oxygenase-1 (HO-1) through the ERK/Nrf2/ARE signaling pathways (Lee et al., 2015).
Stimulation of Insulin Secretion in Mice Islets : this compound stimulates insulin secretion through the cAMP/PKA signaling pathway, presenting a novel approach for diabetes treatment (Hameed et al., 2018).
Anti-melanogenesis and Antigenotoxic Activities : It demonstrates potential as a natural skin depigmenting agent due to its ability to reduce melanogenesis and protect cell DNA against oxidative damage (Imen et al., 2015).
Wide Range of Therapeutic Roles : this compound has multiple pharmacological effects, including antioxidant, anti-inflammatory, anti-cancer, neuroprotective, and cardioprotective properties (Islam et al., 2020).
Inhibition of Cancer Cell Growth : It inhibits the growth of CNE1 human nasopharyngeal cancer cells by targeting the MEK/ERK signaling pathway, inducing autophagy, and suppressing cell migration and invasion (Tang et al., 2020).
Mechanism of Action
Eriodictyol, a flavonoid in the flavanones subclass, is abundantly present in a wide range of medicinal plants, citrus fruits, and vegetables . It has considerable medicinal properties and has been predicted to clarify the mode of action in various cellular and molecular pathways .
Target of Action
This compound has been associated with its ability to modulate a number of cell-signaling cascades . It has been shown to interact with pro-inflammatory cytokines such as tumor necrosis factor (TNF-α), interleukin-6 (IL-6), IL-8, and IL-1β . It also enhances the fork development of O1 (FOXO1) in RA-FLS for rheumatoid arthritis .
Mode of Action
This compound’s interaction with its targets results in a variety of changes. For instance, it has been shown to inhibit glioblastoma migration and invasion by reversing EMT via downregulation of the P38 MAPK/GSK-3β/ZEB1 pathway . It also modulates pro-inflammatory cytokines and enhances FOXO1 .
Biochemical Pathways
This compound affects various cellular and molecular pathways. It has been shown to protect retinal ganglion cells (RGCs) from high glucose-induced oxidative stress and cell apoptosis by activation of the Nrf2/HO-1 pathways . It also inhibits the PI3K/AKT pathway, which plays a crucial role in cell survival and growth .
Pharmacokinetics
The pharmacokinetic parameters of this compound are important to understand its function in the prevention and treatment of diseases . .
Result of Action
This compound has numerous therapeutic roles, including antioxidant, anti-inflammatory, anti-cancer, neuroprotective, cardioprotective, anti-diabetic, anti-obesity, and hepatoprotective effects . It has been reported to maintain homeostasis in organisms by exerting neuroprotective and vascular protective effects .
Action Environment
The action, efficacy, and stability of this compound can be influenced by various environmental factors. For instance, the solubility of this compound can affect its bioavailability and thus its pharmacological effects . .
properties
IUPAC Name |
2-(3,4-dihydroxyphenyl)-5,7-dihydroxy-2,3-dihydrochromen-4-one | |
---|---|---|
Source | PubChem | |
URL | https://pubchem.ncbi.nlm.nih.gov | |
Description | Data deposited in or computed by PubChem | |
InChI |
InChI=1S/C15H12O6/c16-8-4-11(19)15-12(20)6-13(21-14(15)5-8)7-1-2-9(17)10(18)3-7/h1-5,13,16-19H,6H2 | |
Source | PubChem | |
URL | https://pubchem.ncbi.nlm.nih.gov | |
Description | Data deposited in or computed by PubChem | |
InChI Key |
SBHXYTNGIZCORC-UHFFFAOYSA-N | |
Source | PubChem | |
URL | https://pubchem.ncbi.nlm.nih.gov | |
Description | Data deposited in or computed by PubChem | |
Canonical SMILES |
C1C(OC2=CC(=CC(=C2C1=O)O)O)C3=CC(=C(C=C3)O)O | |
Source | PubChem | |
URL | https://pubchem.ncbi.nlm.nih.gov | |
Description | Data deposited in or computed by PubChem | |
Molecular Formula |
C15H12O6 | |
Source | PubChem | |
URL | https://pubchem.ncbi.nlm.nih.gov | |
Description | Data deposited in or computed by PubChem | |
DSSTOX Substance ID |
DTXSID30862178 | |
Record name | (+/-)-Eriodictyol | |
Source | EPA DSSTox | |
URL | https://comptox.epa.gov/dashboard/DTXSID30862178 | |
Description | DSSTox provides a high quality public chemistry resource for supporting improved predictive toxicology. | |
Molecular Weight |
288.25 g/mol | |
Source | PubChem | |
URL | https://pubchem.ncbi.nlm.nih.gov | |
Description | Data deposited in or computed by PubChem | |
Physical Description |
Slightly beige solid; Bland aroma | |
Record name | (\u00b1)-Eriodictyol | |
Source | Joint FAO/WHO Expert Committee on Food Additives (JECFA) | |
URL | https://www.fao.org/food/food-safety-quality/scientific-advice/jecfa/jecfa-flav/details/en/c/2146/ | |
Description | The flavoring agent databse provides the most recent specifications for flavorings evaluated by JECFA. | |
Explanation | Permission from WHO is not required for the use of WHO materials issued under the Creative Commons Attribution-NonCommercial-ShareAlike 3.0 Intergovernmental Organization (CC BY-NC-SA 3.0 IGO) licence. | |
Solubility |
Practically insoluble to insoluble, Sparingly soluble (in ethanol) | |
Record name | (\u00b1)-Eriodictyol | |
Source | Joint FAO/WHO Expert Committee on Food Additives (JECFA) | |
URL | https://www.fao.org/food/food-safety-quality/scientific-advice/jecfa/jecfa-flav/details/en/c/2146/ | |
Description | The flavoring agent databse provides the most recent specifications for flavorings evaluated by JECFA. | |
Explanation | Permission from WHO is not required for the use of WHO materials issued under the Creative Commons Attribution-NonCommercial-ShareAlike 3.0 Intergovernmental Organization (CC BY-NC-SA 3.0 IGO) licence. | |
CAS RN |
4049-38-1 | |
Record name | Eriodictyol, (+/-)- | |
Source | ChemIDplus | |
URL | https://pubchem.ncbi.nlm.nih.gov/substance/?source=chemidplus&sourceid=0004049381 | |
Description | ChemIDplus is a free, web search system that provides access to the structure and nomenclature authority files used for the identification of chemical substances cited in National Library of Medicine (NLM) databases, including the TOXNET system. | |
Record name | (+/-)-Eriodictyol | |
Source | EPA DSSTox | |
URL | https://comptox.epa.gov/dashboard/DTXSID30862178 | |
Description | DSSTox provides a high quality public chemistry resource for supporting improved predictive toxicology. | |
Record name | (+/-)-Eriodictyol | |
Source | European Chemicals Agency (ECHA) | |
URL | https://echa.europa.eu/information-on-chemicals | |
Description | The European Chemicals Agency (ECHA) is an agency of the European Union which is the driving force among regulatory authorities in implementing the EU's groundbreaking chemicals legislation for the benefit of human health and the environment as well as for innovation and competitiveness. | |
Explanation | Use of the information, documents and data from the ECHA website is subject to the terms and conditions of this Legal Notice, and subject to other binding limitations provided for under applicable law, the information, documents and data made available on the ECHA website may be reproduced, distributed and/or used, totally or in part, for non-commercial purposes provided that ECHA is acknowledged as the source: "Source: European Chemicals Agency, http://echa.europa.eu/". Such acknowledgement must be included in each copy of the material. ECHA permits and encourages organisations and individuals to create links to the ECHA website under the following cumulative conditions: Links can only be made to webpages that provide a link to the Legal Notice page. | |
Record name | ERIODICTYOL, (±)- | |
Source | FDA Global Substance Registration System (GSRS) | |
URL | https://gsrs.ncats.nih.gov/ginas/app/beta/substances/CQT975GLYF | |
Description | The FDA Global Substance Registration System (GSRS) enables the efficient and accurate exchange of information on what substances are in regulated products. Instead of relying on names, which vary across regulatory domains, countries, and regions, the GSRS knowledge base makes it possible for substances to be defined by standardized, scientific descriptions. | |
Explanation | Unless otherwise noted, the contents of the FDA website (www.fda.gov), both text and graphics, are not copyrighted. They are in the public domain and may be republished, reprinted and otherwise used freely by anyone without the need to obtain permission from FDA. Credit to the U.S. Food and Drug Administration as the source is appreciated but not required. | |
Retrosynthesis Analysis
AI-Powered Synthesis Planning: Our tool employs the Template_relevance Pistachio, Template_relevance Bkms_metabolic, Template_relevance Pistachio_ringbreaker, Template_relevance Reaxys, Template_relevance Reaxys_biocatalysis model, leveraging a vast database of chemical reactions to predict feasible synthetic routes.
One-Step Synthesis Focus: Specifically designed for one-step synthesis, it provides concise and direct routes for your target compounds, streamlining the synthesis process.
Accurate Predictions: Utilizing the extensive PISTACHIO, BKMS_METABOLIC, PISTACHIO_RINGBREAKER, REAXYS, REAXYS_BIOCATALYSIS database, our tool offers high-accuracy predictions, reflecting the latest in chemical research and data.
Strategy Settings
Precursor scoring | Relevance Heuristic |
---|---|
Min. plausibility | 0.01 |
Model | Template_relevance |
Template Set | Pistachio/Bkms_metabolic/Pistachio_ringbreaker/Reaxys/Reaxys_biocatalysis |
Top-N result to add to graph | 6 |
Feasible Synthetic Routes
Q & A
Q1: What is the primary mechanism of action of eriodictyol in exerting its anticancer effects?
A1: this compound has been shown to exhibit anticancer activity against various cancer cell lines, including gastric cancer [], glioma [], and lung cancer []. One of its primary mechanisms involves the inhibition of the PI3K/AKT signaling pathway [, , ]. This pathway plays a crucial role in cell growth, proliferation, and survival, and its dysregulation is frequently observed in various cancers. By suppressing this pathway, this compound induces apoptosis (programmed cell death) in cancer cells, thereby inhibiting their growth and proliferation.
Q2: How does this compound interact with the PI3K/AKT pathway?
A2: While the precise binding site of this compound on PI3K/AKT remains to be fully elucidated, studies suggest that it directly interacts with components of this pathway, leading to the inhibition of downstream signaling. Molecular docking simulations have demonstrated favorable binding energies for this compound with PI3K and AKT [], supporting the notion of a direct interaction.
Q3: Beyond PI3K/AKT, what other signaling pathways are modulated by this compound?
A3: this compound's influence extends to other crucial signaling pathways. It has been observed to activate the Nrf2/HO-1 pathway, a critical cellular defense mechanism against oxidative stress [, , , ]. Additionally, this compound downregulates the NF-κB pathway, a key regulator of inflammation [, , ]. These findings highlight this compound’s multi-targeted approach to combating various pathological conditions.
Q4: this compound has shown potential in preclinical models of Alzheimer’s disease. What mechanisms contribute to this neuroprotective effect?
A4: this compound demonstrates promising neuroprotective properties in experimental models of Alzheimer's disease [, , ]. It can reduce amyloid-β (Aβ) aggregation, a hallmark of the disease, and alleviate tau hyperphosphorylation, another pathological feature linked to neuronal dysfunction. Furthermore, this compound has been shown to inhibit ferroptosis, a form of regulated cell death implicated in neurodegeneration, by activating the Nrf2/HO-1 signaling pathway in a vitamin D receptor (VDR)-dependent manner [].
Q5: How does this compound influence oxidative stress, and what are the implications for its therapeutic potential?
A5: this compound exhibits potent antioxidant activity through multiple mechanisms. It acts as a scavenger of reactive oxygen species (ROS) [], directly neutralizing these harmful molecules. Moreover, this compound enhances the activity and expression of antioxidant enzymes, including superoxide dismutase (SOD), catalase (CAT), and glutathione peroxidase (GPx) [, , , ]. This multi-faceted antioxidant capacity contributes to its protective effects in various disease models characterized by oxidative damage, such as acute lung injury [], diabetic nephropathy [], and neurodegenerative diseases [, ].
Q6: What is the molecular formula and weight of this compound?
A6: The molecular formula of this compound is C15H12O6, and its molecular weight is 288.25 g/mol.
Q7: Are there any studies investigating the relationship between the structure of this compound and its biological activity?
A7: Yes, studies have shown that the number of hydroxyl groups on the B-ring of flavonoids, including this compound, significantly affects their antioxidant activity and interaction with the C1B domain of PKCδ []. This compound, with two hydroxyl groups on its B-ring, exhibits higher antioxidant activity than pinocembrin and naringenin, which have one and no hydroxyl group on their B-ring, respectively.
Q8: What is known about the pharmacokinetics of this compound?
A8: this compound, when administered orally, is absorbed and metabolized, primarily into glucuronide conjugates. The solubility and vehicle used for administration significantly influence its pharmacokinetic profile []. Research suggests that this compound's bioavailability might be enhanced by co-administration with UGT1A inhibitors such as glycyrrhetinic acid [].
Disclaimer and Information on In-Vitro Research Products
Please be aware that all articles and product information presented on BenchChem are intended solely for informational purposes. The products available for purchase on BenchChem are specifically designed for in-vitro studies, which are conducted outside of living organisms. In-vitro studies, derived from the Latin term "in glass," involve experiments performed in controlled laboratory settings using cells or tissues. It is important to note that these products are not categorized as medicines or drugs, and they have not received approval from the FDA for the prevention, treatment, or cure of any medical condition, ailment, or disease. We must emphasize that any form of bodily introduction of these products into humans or animals is strictly prohibited by law. It is essential to adhere to these guidelines to ensure compliance with legal and ethical standards in research and experimentation.