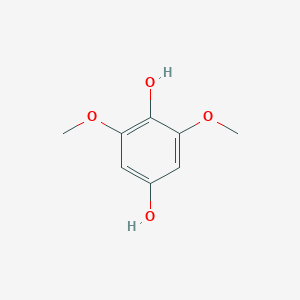
2,6-dimethoxybenzene-1,4-diol
Overview
Description
Preparation Methods
Methylation of Hydroquinone
Reaction Mechanism and Standard Protocol
The most widely documented synthesis of 2,6-dimethoxybenzene-1,4-diol involves the methylation of hydroquinone (1,4-dihydroxybenzene). This two-step process selectively substitutes hydroxyl groups at the 2 and 6 positions using methylating agents such as dimethyl sulfate or methyl iodide. The reaction is typically conducted in the presence of a base, such as potassium carbonate (K₂CO₃), to deprotonate the hydroxyl groups and facilitate nucleophilic substitution .
The general reaction sequence is as follows:
-
First Methylation :
Hydroquinone reacts with one equivalent of dimethyl sulfate under reflux conditions (80–100°C) in a polar aprotic solvent like acetone or dimethylformamide (DMF). This step preferentially substitutes one hydroxyl group, yielding 2-methoxyhydroquinone. -
Second Methylation :
A second equivalent of dimethyl sulfate is introduced to substitute the remaining hydroxyl group at the 6 position. The reaction mixture is maintained under reflux for 6–12 hours to ensure complete conversion .
Optimization of Reaction Conditions
Critical parameters influencing yield and selectivity include:
Parameter | Optimal Range | Impact on Yield |
---|---|---|
Temperature | 80–100°C | Higher temperatures accelerate reaction but risk over-methylation |
Solvent | Acetone or DMF | Polar aprotic solvents enhance reagent solubility |
Base | K₂CO₃ (2.5 equiv) | Excess base minimizes side reactions |
Methylating Agent | Dimethyl sulfate (2.2 equiv) | Stoichiometric excess ensures complete substitution |
Under these conditions, yields of 70–85% are routinely achieved . Side products, such as over-methylated derivatives (e.g., 1,2,4-trimethoxybenzene), are minimized by controlling the stoichiometry of the methylating agent.
Catalytic Reductive Demethoxylation of Lignin-Derived Precursors
Lignin as a Feedstock for this compound
Recent studies have explored lignin depolymerization products as sustainable precursors for this compound. Beech lignin-derived 2,6-dimethoxybenzoquinone (DMBQ) is hydrogenated using RANEY® Ni catalysts to produce 1,4-cyclohexanediol (14CHDO) and subsequently this compound via selective demethoxylation .
Hydrogenation and Demethoxylation Protocol
The catalytic transformation involves two stages:
-
Hydrogenation of DMBQ :
DMBQ is subjected to hydrogenation at 170°C under 30 bar H₂ pressure in methanol, using RANEY® Ni. This step reduces the quinone to this compound with 86.5% molar yield . -
Demethoxylation :
Further treatment with NiMn catalysts under hydrogen-free conditions (isopropanol as a hydrogen donor) removes methoxy groups, yielding 1,4-cyclohexanediol. Adjusting the Mn content in the catalyst enhances selectivity for demethoxylation over competing pathways .
Catalyst Design and Performance
Modification of RANEY® Ni with manganese (NiMn) significantly improves activity and stability. Key characteristics of the NiMn catalyst include:
Property | NiMn (5 wt% Mn) | RANEY® Ni |
---|---|---|
Surface Area (BET) | 98 m²/g | 45 m²/g |
Turnover Frequency | 0.42 min⁻¹ | 0.28 min⁻¹ |
Yield of 14CHDO | 86.1% | 78.3% |
The NiMn alloy structure, confirmed by XRD and XPS, facilitates electron transfer between Ni and Mn, enhancing hydrogenation efficiency .
Comparative Analysis of Preparation Methods
Efficiency and Sustainability
A side-by-side comparison reveals trade-offs between traditional methylation and catalytic reductive methods:
Metric | Methylation Method | Catalytic Reductive Method |
---|---|---|
Yield | 70–85% | 86–90% |
Reaction Time | 8–12 hours | 6–8 hours |
Temperature | 80–100°C | 160–200°C |
Solvent | DMF/Acetone | Isopropanol/Methanol |
Carbon Footprint | Higher (fossil-derived reagents) | Lower (biomass feedstock) |
The catalytic approach offers superior yields and sustainability but requires specialized equipment for high-pressure hydrogenation .
Industrial Scalability
While methylation remains the industry standard due to its simplicity, lignin-based methods are gaining traction in biorefineries. For example, a pilot-scale study achieved 10.7 wt% this compound from lignin dimers using continuous-flow hydrogenation reactors .
Chemical Reactions Analysis
Types of Reactions: 2,6-dimethoxybenzene-1,4-diol undergoes various chemical reactions, including oxidation, reduction, and substitution.
Common Reagents and Conditions:
Oxidation: It can be oxidized using reagents such as potassium permanganate or hydrogen peroxide, leading to the formation of quinones.
Reduction: Reduction reactions can be carried out using reducing agents like sodium borohydride, resulting in the formation of hydroquinone derivatives.
Major Products: The major products formed from these reactions include various quinones, hydroquinone derivatives, and substituted phenols .
Scientific Research Applications
Pharmaceutical Applications
1. Precursor for Drug Synthesis
2,6-Dimethoxybenzene-1,4-diol serves as an intermediate in the synthesis of various pharmaceutical compounds. For instance, it can be transformed into more complex structures that exhibit biological activity. One notable application is its conversion to 1,4-cyclohexanediol through environmentally friendly catalytic processes. This compound is used in the production of analgesics like cebranopadol .
2. Antioxidant Properties
Research has indicated that compounds similar to this compound possess antioxidant properties. These properties are crucial in developing drugs aimed at reducing oxidative stress-related diseases .
Material Science Applications
1. Polymer Production
The compound is utilized in the synthesis of polymers that require specific functional groups for enhanced properties. For example, it can act as a building block for producing scratch-resistant coatings and other advanced materials .
2. Lignin Valorization
Recent studies have explored the use of lignin-derived this compound in sustainable material production. The transformation of lignin into valuable chemicals via catalytic processes highlights its potential in developing renewable materials .
Environmental Applications
1. Biodegradation Studies
The compound has been investigated for its role in biodegradation processes. Certain fungi can degrade lignin compounds into simpler phenolic structures like this compound. This aspect is significant for bioremediation efforts aimed at breaking down complex organic pollutants .
2. Inhibitory Effects in Biomass Conversion
In biomass conversion processes, this compound has been identified as an inhibitor affecting microbial activity. Understanding its inhibitory effects can help optimize conditions for energy generation from biomass .
Data Table: Applications Overview
Case Studies
Case Study 1: Production of 1,4-Cyclohexanediol
A study demonstrated the conversion of lignin-derived 2,6-dimethoxybenzoquinone to 1,4-cyclohexanediol using Raney NiMn catalysts under hydrogen-free conditions. This method achieved a yield of up to 86% and represents a significant advancement in sustainable chemical production from renewable resources .
Case Study 2: Biodegradation by Abortiporus biennis
Research indicated that the fungus Abortiporus biennis can degrade coniferyl alcohol into vanillin and coniferyl aldehyde while also producing this compound as an intermediate product. This showcases the compound's role in natural degradation processes and its potential application in bioremediation strategies .
Mechanism of Action
The mechanism of action of 2,6-dimethoxyhydroquinone involves its ability to generate reactive oxygen species (ROS) such as hydrogen peroxide and hydroxyl radicals. These ROS can induce oxidative stress in cells, leading to cytotoxic effects. The compound’s cytotoxicity is enhanced in the presence of L-ascorbic acid, which synergistically increases the production of ROS . This mechanism is particularly relevant in its potential anti-cancer applications, where the generated ROS can damage tumor cells.
Comparison with Similar Compounds
Chemical Identity :
- IUPAC Name : 2,6-Dimethoxybenzene-1,4-diol
- Molecular Formula : C₈H₁₀O₄
- Molecular Weight : 170.16 g/mol
- CAS Number : 15233-65-5
- Synonyms: 1,4-Dihydroxy-2,6-dimethoxybenzene; 2,6-Dimethoxyhydroquinone .
Physicochemical Properties :
- Density : 1.267 g/cm³
- Melting Point : 158–162 °C
- Boiling Point : 352.9 °C (at 760 mmHg)
- LogP : 1.115 (indicating moderate hydrophobicity)
Synthesis and Applications: this compound (hereafter referred to as 1A) is a key intermediate in lignin valorization. It is produced via catalytic hydrogenation of lignin-derived compounds using RANEY® Ni or Ni-Mn catalysts. Solvent choice (e.g., methanol vs. ethanol) and temperature critically influence its formation. For instance, methanol favors 1A, while ethanol promotes demethoxylation to yield cyclohexanediols (CHDO) . Applications include its role as a precursor for CHDO, which is valuable in polymer production, and its involvement in lignin biodegradation pathways via microbial oxidoreductases .
Comparison with Structurally Similar Compounds
Substituent-Based Comparison
The table below compares 1A with analogs differing in substituents or functional groups:
Compound Name | Molecular Formula | Substituents | Molecular Weight | Melting Point (°C) | Key Applications |
---|---|---|---|---|---|
This compound | C₈H₁₀O₄ | 2,6-OCH₃; 1,4-OH | 170.16 | 158–162 | Lignin conversion; CHDO precursor |
2,6-Difluorobenzene-1,4-diol | C₆H₄F₂O₂ | 2,6-F; 1,4-OH | 146.09 | Not reported | Pharmaceutical intermediates |
2-Chlorobenzene-1,4-diol | C₆H₅ClO₂ | 2-Cl; 1,4-OH | 144.55 | Not reported | Electronic structure studies |
2,5-Dimethoxybenzene-1,4-dicarbaldehyde | C₁₀H₁₀O₄ | 2,5-OCH₃; 1,4-CHO | 194.18 | Not reported | Organic synthesis building block |
Key Observations :
- Electron-Withdrawing vs. Electron-Donating Groups : Fluorine and chlorine substituents (electron-withdrawing) reduce electron density on the aromatic ring compared to methoxy groups (electron-donating), altering reactivity in hydrogenation and oxidation reactions .
- Biological Activity : Pyrimidine derivatives with thioether substituents (e.g., 2,6-bis(4,6-dimethylpyrimidin-2-ylthio)benzene-1,4-diol) exhibit antimicrobial activity, suggesting that sulfur-containing analogs may have enhanced bioactivity compared to 1A .
Reaction Behavior and Catalytic Pathways
- Hydrogenation: At 160°C, 1A is the dominant product during lignin hydrogenation. At 180–200°C, demethoxylation occurs, yielding CHDO. Above 220°C, over-reaction produces cyclohexanol .
- Solvent Effects: Methanol stabilizes 1A due to strong adsorption of methoxide ions on RANEY® Ni, suppressing demethoxylation. Ethanol allows partial demethoxylation, highlighting solvent polarity’s role in reaction selectivity .
Electronic and Spectroscopic Properties
- UV Spectroscopy: Chlorinated derivatives (e.g., 2-chlorobenzene-1,4-diol) exhibit distinct UV absorption profiles due to altered π→π* transitions, whereas 1A’s methoxy groups result in redshifted spectra compared to unsubstituted hydroquinone .
- Thermal Stability : 1A ’s higher melting point (158–162°C) compared to 2,6-difluorobenzene-1,4-diol (data unavailable) reflects stronger intermolecular hydrogen bonding from methoxy groups .
Research Findings and Industrial Relevance
- Lignin Valorization : 1A is a critical intermediate in sustainable chemical production. Catalytic systems like Ni-Mn achieve 86% yield of CHDO from 1A under optimized conditions (200°C, 1 hour) .
- Microbial Degradation: Bacillus species oxidize 2,6-dimethoxyphenol to 1A, linking its production to ligninolytic enzyme activity (e.g., copA gene products) .
- Safety and Handling : 1A requires storage in dry, ventilated conditions to prevent decomposition, contrasting with more stable derivatives like 1,4-dimethoxybenzene, which lacks hydroxyl groups .
Biological Activity
Antioxidant Properties
2,6-Dimethoxybenzene-1,4-diol exhibits significant antioxidant activity. Hydroquinones are known for their ability to scavenge free radicals and reduce oxidative stress in biological systems. This property is crucial in preventing cellular damage caused by reactive oxygen species (ROS), which are implicated in various diseases including cancer and neurodegenerative disorders .
Anticancer Activity
Research has indicated that derivatives of hydroquinones, including this compound, may possess anticancer properties. A study tested the cytotoxic effects of various hydroquinone derivatives on cancer cell lines using the MTS assay. The results suggested that modifications to the hydroquinone structure could enhance its anticancer efficacy .
Antifungal Activity
In a study focusing on the synthesis of phenolic compounds for agricultural applications, this compound was evaluated for its antifungal properties against Botrytis cinerea, a common plant pathogen. The compound demonstrated notable fungicidal activity, suggesting its potential use as a natural fungicide in horticulture .
Biochemical Mechanisms
The biological mechanisms underlying the activity of this compound include:
- Reduction of oxidative stress : By acting as an electron donor in redox reactions.
- Inhibition of tumor growth : Through modulation of signaling pathways involved in cell proliferation and apoptosis.
- Antimicrobial action : By disrupting cellular membranes or inhibiting key metabolic pathways in pathogens .
Study on Antioxidant Activity
A recent investigation assessed the antioxidant capacity of various hydroquinones including this compound using DPPH radical scavenging assays. The results indicated a strong correlation between the number of hydroxyl groups and antioxidant activity. The compound demonstrated effective scavenging ability comparable to well-known antioxidants like ascorbic acid.
Application in Agriculture
Another study explored the use of this compound as a natural pesticide. When applied to crops affected by Botrytis cinerea, it significantly reduced fungal infection rates by over 60%, showcasing its potential as an eco-friendly alternative to synthetic fungicides .
Data Table: Biological Activities of this compound
Q & A
Basic Research Questions
Q. What are the standard synthetic routes for 2,6-dimethoxybenzene-1,4-diol, and how do reaction conditions influence yield and purity?
Methodological Answer: The compound is typically synthesized via electrophilic substitution or alkylation of hydroquinone derivatives. For example:
- Methoxy group introduction : Alkylation of 1,4-dihydroxybenzene with methyl iodide in the presence of a base (e.g., K₂CO₃) under reflux conditions. Excess methylating agents may lead to over-alkylation, requiring careful stoichiometric control .
- Crystal structure optimization : Evidence from benzobisoxazole synthesis (e.g., 2,6-dithiophene derivatives) highlights the importance of solvent choice (polar aprotic solvents like DMF) and temperature (60–80°C) for achieving high crystallinity .
Key Data : Yields range from 50–75% depending on purification methods (e.g., column chromatography vs. recrystallization) .
Q. How can researchers characterize the structural and electronic properties of this compound?
Methodological Answer:
- Spectroscopic techniques :
- ¹H/¹³C NMR : Methoxy protons resonate at δ 3.8–4.0 ppm, while aromatic protons appear as singlet(s) due to symmetry (δ 6.5–7.0 ppm) .
- FT-IR : O–H stretching (3200–3400 cm⁻¹), C–O–C (1250 cm⁻¹), and aromatic C=C (1500–1600 cm⁻¹) .
- X-ray crystallography : Symmetrical methoxy substitution results in planar geometry, with intermolecular hydrogen bonding influencing crystal packing .
Q. What are the common oxidative pathways for this compound, and how do they affect downstream applications?
Methodological Answer:
- Quinone formation : Oxidation with KMnO₄ or H₂O₂ in acidic media converts the diol to 2,6-dimethoxy-1,4-benzoquinone. This reaction is pH-sensitive; excess acid may degrade the quinone product .
- Stability considerations : The methoxy groups stabilize the aromatic ring against over-oxidation, but prolonged exposure to strong oxidants (e.g., CrO₃) can cleave the ring .
Advanced Research Questions
Q. How can computational modeling (e.g., DFT) predict the reactivity of this compound in catalytic systems?
Methodological Answer:
- DFT studies : Calculate frontier molecular orbitals (HOMO/LUMO) to predict sites for electrophilic attack. Methoxy groups act as electron donors, lowering the energy barrier for reactions at the para positions .
- Docking simulations : Used to study interactions with enzymes (e.g., laccases) for biodegradation or polymerization studies. Methoxy groups may hinder binding due to steric effects .
Q. How do conflicting data on redox reversibility in diol-quinone systems arise, and how can they be resolved experimentally?
Methodological Answer:
- Contradiction source : Redox reversibility depends on substituent electron effects. While 2,6-dimethoxy derivatives show reversible interconversion under mild conditions (e.g., p-benzoquinone/H₂), harsher conditions (e.g., strong acids) lead to irreversible degradation .
- Resolution strategy :
Q. What strategies optimize the Heck-Matsuda reaction for functionalizing this compound in flow reactors?
Methodological Answer:
- Ligand-free catalysis : Flow reactors enhance reaction kinetics, enabling Pd-catalyzed couplings without ligands (unlike batch systems). Key parameters:
- Residence time : 10–15 minutes at 50°C.
- Solvent : MeOH improves solubility and reduces Pd nanoparticle formation .
Data : Conversions ≥90% achieved in flow vs. ≤70% in batch .
Properties
IUPAC Name |
2,6-dimethoxybenzene-1,4-diol | |
---|---|---|
Source | PubChem | |
URL | https://pubchem.ncbi.nlm.nih.gov | |
Description | Data deposited in or computed by PubChem | |
InChI |
InChI=1S/C8H10O4/c1-11-6-3-5(9)4-7(12-2)8(6)10/h3-4,9-10H,1-2H3 | |
Source | PubChem | |
URL | https://pubchem.ncbi.nlm.nih.gov | |
Description | Data deposited in or computed by PubChem | |
InChI Key |
GXAVBFNRWXCOPY-UHFFFAOYSA-N | |
Source | PubChem | |
URL | https://pubchem.ncbi.nlm.nih.gov | |
Description | Data deposited in or computed by PubChem | |
Canonical SMILES |
COC1=CC(=CC(=C1O)OC)O | |
Source | PubChem | |
URL | https://pubchem.ncbi.nlm.nih.gov | |
Description | Data deposited in or computed by PubChem | |
Molecular Formula |
C8H10O4 | |
Source | PubChem | |
URL | https://pubchem.ncbi.nlm.nih.gov | |
Description | Data deposited in or computed by PubChem | |
DSSTOX Substance ID |
DTXSID40165001 | |
Record name | 2,6-Dimethoxyhydroquinone | |
Source | EPA DSSTox | |
URL | https://comptox.epa.gov/dashboard/DTXSID40165001 | |
Description | DSSTox provides a high quality public chemistry resource for supporting improved predictive toxicology. | |
Molecular Weight |
170.16 g/mol | |
Source | PubChem | |
URL | https://pubchem.ncbi.nlm.nih.gov | |
Description | Data deposited in or computed by PubChem | |
CAS No. |
15233-65-5 | |
Record name | 2,6-Dimethoxyhydroquinone | |
Source | CAS Common Chemistry | |
URL | https://commonchemistry.cas.org/detail?cas_rn=15233-65-5 | |
Description | CAS Common Chemistry is an open community resource for accessing chemical information. Nearly 500,000 chemical substances from CAS REGISTRY cover areas of community interest, including common and frequently regulated chemicals, and those relevant to high school and undergraduate chemistry classes. This chemical information, curated by our expert scientists, is provided in alignment with our mission as a division of the American Chemical Society. | |
Explanation | The data from CAS Common Chemistry is provided under a CC-BY-NC 4.0 license, unless otherwise stated. | |
Record name | 2,6-Dimethoxyhydroquinone | |
Source | ChemIDplus | |
URL | https://pubchem.ncbi.nlm.nih.gov/substance/?source=chemidplus&sourceid=0015233655 | |
Description | ChemIDplus is a free, web search system that provides access to the structure and nomenclature authority files used for the identification of chemical substances cited in National Library of Medicine (NLM) databases, including the TOXNET system. | |
Record name | 2,6-Dimethoxyhydroquinone | |
Source | DTP/NCI | |
URL | https://dtp.cancer.gov/dtpstandard/servlet/dwindex?searchtype=NSC&outputformat=html&searchlist=49356 | |
Description | The NCI Development Therapeutics Program (DTP) provides services and resources to the academic and private-sector research communities worldwide to facilitate the discovery and development of new cancer therapeutic agents. | |
Explanation | Unless otherwise indicated, all text within NCI products is free of copyright and may be reused without our permission. Credit the National Cancer Institute as the source. | |
Record name | 2,6-Dimethoxyhydroquinone | |
Source | EPA DSSTox | |
URL | https://comptox.epa.gov/dashboard/DTXSID40165001 | |
Description | DSSTox provides a high quality public chemistry resource for supporting improved predictive toxicology. | |
Record name | 2,6-dimethoxyhydroquinone | |
Source | European Chemicals Agency (ECHA) | |
URL | https://echa.europa.eu/substance-information/-/substanceinfo/100.035.696 | |
Description | The European Chemicals Agency (ECHA) is an agency of the European Union which is the driving force among regulatory authorities in implementing the EU's groundbreaking chemicals legislation for the benefit of human health and the environment as well as for innovation and competitiveness. | |
Explanation | Use of the information, documents and data from the ECHA website is subject to the terms and conditions of this Legal Notice, and subject to other binding limitations provided for under applicable law, the information, documents and data made available on the ECHA website may be reproduced, distributed and/or used, totally or in part, for non-commercial purposes provided that ECHA is acknowledged as the source: "Source: European Chemicals Agency, http://echa.europa.eu/". Such acknowledgement must be included in each copy of the material. ECHA permits and encourages organisations and individuals to create links to the ECHA website under the following cumulative conditions: Links can only be made to webpages that provide a link to the Legal Notice page. | |
Record name | 2,6-DIMETHOXYHYDROQUINONE | |
Source | FDA Global Substance Registration System (GSRS) | |
URL | https://gsrs.ncats.nih.gov/ginas/app/beta/substances/9U2C06A3MM | |
Description | The FDA Global Substance Registration System (GSRS) enables the efficient and accurate exchange of information on what substances are in regulated products. Instead of relying on names, which vary across regulatory domains, countries, and regions, the GSRS knowledge base makes it possible for substances to be defined by standardized, scientific descriptions. | |
Explanation | Unless otherwise noted, the contents of the FDA website (www.fda.gov), both text and graphics, are not copyrighted. They are in the public domain and may be republished, reprinted and otherwise used freely by anyone without the need to obtain permission from FDA. Credit to the U.S. Food and Drug Administration as the source is appreciated but not required. | |
Retrosynthesis Analysis
AI-Powered Synthesis Planning: Our tool employs the Template_relevance Pistachio, Template_relevance Bkms_metabolic, Template_relevance Pistachio_ringbreaker, Template_relevance Reaxys, Template_relevance Reaxys_biocatalysis model, leveraging a vast database of chemical reactions to predict feasible synthetic routes.
One-Step Synthesis Focus: Specifically designed for one-step synthesis, it provides concise and direct routes for your target compounds, streamlining the synthesis process.
Accurate Predictions: Utilizing the extensive PISTACHIO, BKMS_METABOLIC, PISTACHIO_RINGBREAKER, REAXYS, REAXYS_BIOCATALYSIS database, our tool offers high-accuracy predictions, reflecting the latest in chemical research and data.
Strategy Settings
Precursor scoring | Relevance Heuristic |
---|---|
Min. plausibility | 0.01 |
Model | Template_relevance |
Template Set | Pistachio/Bkms_metabolic/Pistachio_ringbreaker/Reaxys/Reaxys_biocatalysis |
Top-N result to add to graph | 6 |
Feasible Synthetic Routes
Disclaimer and Information on In-Vitro Research Products
Please be aware that all articles and product information presented on BenchChem are intended solely for informational purposes. The products available for purchase on BenchChem are specifically designed for in-vitro studies, which are conducted outside of living organisms. In-vitro studies, derived from the Latin term "in glass," involve experiments performed in controlled laboratory settings using cells or tissues. It is important to note that these products are not categorized as medicines or drugs, and they have not received approval from the FDA for the prevention, treatment, or cure of any medical condition, ailment, or disease. We must emphasize that any form of bodily introduction of these products into humans or animals is strictly prohibited by law. It is essential to adhere to these guidelines to ensure compliance with legal and ethical standards in research and experimentation.