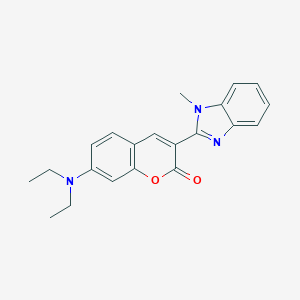
Coumarin 30
Overview
Description
Coumarin 30 is a synthetic organic compound belonging to the coumarin family, which is characterized by a benzopyrone structure. Coumarins are known for their diverse biological activities and applications in various fields, including medicine, agriculture, and industry. This compound, in particular, is widely used as a fluorescent dye due to its excellent photophysical properties.
Mechanism of Action
Target of Action
Coumarin primarily targets the Cytochrome P450 2A6 enzyme in humans . This enzyme plays a crucial role in the metabolism of various drugs and xenobiotics . In addition, coumarin has been identified as a promising pharmacophore for the development of novel anticancer drugs .
Mode of Action
Coumarin inhibits the synthesis of vitamin K , a key component in blood clotting . It interacts with its targets, leading to changes in their function. For instance, it has been shown to inhibit the active form of the GPIIb/IIIa complex on the membrane of platelets, thus preventing platelet aggregation .
Biochemical Pathways
Coumarin affects the phenylpropanoid pathway in plants . It is involved in the ortho-hydroxylation of hydroxycinnamic acids, a pivotal step in the biosynthesis of simple coumarin, umbelliferone, and other hydroxylated coumarins . Multiple P450 enzymes are also involved in furanocoumarin synthesis, a major class of phytoalexins derived from umbelliferone .
Pharmacokinetics
It is known that the addition of a glucuronic acid molecule to 7-hydroxycoumarin forms a more water-soluble compound, which is readily excreted from the body in urine .
Result of Action
The molecular and cellular effects of coumarin’s action are diverse. It has been found to have anticoagulant effects due to its inhibition of vitamin K synthesis . Additionally, coumarin derivatives have shown potential as anticancer agents . For instance, they have been found to exhibit remarkable antimitotic effects against certain cancer cell lines .
Action Environment
The action, efficacy, and stability of coumarin can be influenced by various environmental factors. For instance, its fluorescence behavior upon excitation with ultraviolet (UV) light makes it a versatile fluorophore with wide-ranging applications in science and technology . This property allows coumarin to play a key role in fluorescent labeling of biomolecules, metal ion detection, microenvironment polarity detection, and pH detection .
Biochemical Analysis
Biochemical Properties
Coumarin 30 plays a significant role in biochemical reactions, particularly in the inhibition of certain enzymes. It interacts with enzymes such as cytochrome P450, which is involved in the metabolism of various substances in the liver . This compound inhibits the activity of cytochrome P450, leading to altered metabolic processes. Additionally, this compound has been shown to interact with proteins involved in cell signaling pathways, such as protein kinases, which regulate various cellular functions .
Cellular Effects
This compound exerts various effects on different types of cells and cellular processes. It has been observed to inhibit the growth of cancer cells by inducing apoptosis, or programmed cell death . This compound also affects cell signaling pathways, such as the PI3K/AKT/mTOR pathway, which is crucial for cell survival and proliferation . Furthermore, this compound influences gene expression by modulating the activity of transcription factors, leading to changes in cellular metabolism and function .
Molecular Mechanism
The molecular mechanism of action of this compound involves its binding interactions with biomolecules and enzyme inhibition. This compound binds to the active site of cytochrome P450 enzymes, inhibiting their activity and altering the metabolism of various substrates . It also interacts with protein kinases, leading to the inhibition of cell signaling pathways and subsequent changes in gene expression . These interactions result in the modulation of cellular processes, such as apoptosis and cell proliferation .
Temporal Effects in Laboratory Settings
In laboratory settings, the effects of this compound have been observed to change over time. Studies have shown that this compound is relatively stable under standard laboratory conditions, with minimal degradation over time . Prolonged exposure to this compound can lead to changes in cellular function, such as increased apoptosis and altered gene expression . These long-term effects have been observed in both in vitro and in vivo studies .
Dosage Effects in Animal Models
The effects of this compound vary with different dosages in animal models. At low doses, this compound has been shown to have minimal toxic effects and can inhibit the growth of cancer cells . At high doses, this compound can cause adverse effects, such as liver toxicity and damage to other organs . Threshold effects have been observed, where the therapeutic benefits of this compound are maximized at specific dosages, while higher doses lead to toxicity .
Metabolic Pathways
This compound is involved in various metabolic pathways, including its metabolism by cytochrome P450 enzymes . The primary metabolic pathway involves the hydroxylation of this compound, followed by conjugation reactions that facilitate its excretion from the body . This compound also interacts with other enzymes and cofactors involved in metabolic processes, leading to changes in metabolite levels and metabolic flux .
Transport and Distribution
This compound is transported and distributed within cells and tissues through various mechanisms. It interacts with transporters and binding proteins that facilitate its uptake and distribution . This compound has been shown to accumulate in specific tissues, such as the liver, where it exerts its effects on metabolic processes . The localization and accumulation of this compound within cells are influenced by its interactions with transport proteins and cellular structures .
Subcellular Localization
The subcellular localization of this compound plays a crucial role in its activity and function. This compound has been observed to localize within the endoplasmic reticulum, where it interacts with cytochrome P450 enzymes . This localization is facilitated by targeting signals and post-translational modifications that direct this compound to specific cellular compartments . The activity of this compound is influenced by its subcellular localization, as it modulates the function of enzymes and other biomolecules within these compartments .
Preparation Methods
Synthetic Routes and Reaction Conditions
Coumarin 30 can be synthesized through several methods, with the Pechmann condensation being one of the most common. This reaction involves the condensation of phenols with β-ketoesters in the presence of a strong acid catalyst, such as sulfuric acid or aluminum chloride. The reaction typically proceeds under reflux conditions, yielding coumarin derivatives.
Another method involves the Knoevenagel condensation, where aromatic aldehydes react with active methylene compounds in the presence of a base, such as piperidine or pyridine. This reaction is often carried out under mild conditions and provides high yields of coumarin derivatives.
Industrial Production Methods
Industrial production of this compound often involves large-scale Pechmann or Knoevenagel condensations, utilizing continuous flow reactors to ensure consistent product quality and yield. The use of green chemistry principles, such as solvent-free conditions and recyclable catalysts, is also being explored to make the production process more environmentally friendly.
Chemical Reactions Analysis
Types of Reactions
Coumarin 30 undergoes various chemical reactions, including:
Oxidation: this compound can be oxidized to form coumarin-3-carboxylic acid using oxidizing agents like potassium permanganate or chromium trioxide.
Reduction: Reduction of this compound can yield dihydrocoumarin derivatives, typically using reducing agents such as sodium borohydride or lithium aluminum hydride.
Substitution: Electrophilic substitution reactions can introduce various functional groups into the coumarin ring, enhancing its chemical diversity. Common reagents include halogens, nitrating agents, and sulfonating agents.
Common Reagents and Conditions
Oxidation: Potassium permanganate in aqueous solution, chromium trioxide in acetic acid.
Reduction: Sodium borohydride in ethanol, lithium aluminum hydride in tetrahydrofuran.
Substitution: Halogens (chlorine, bromine) in the presence of Lewis acids, nitrating agents (nitric acid) in sulfuric acid, sulfonating agents (sulfuric acid) under reflux.
Major Products Formed
Oxidation: Coumarin-3-carboxylic acid.
Reduction: Dihydrocoumarin derivatives.
Substitution: Halogenated, nitrated, and sulfonated coumarin derivatives.
Scientific Research Applications
Coumarin 30 has a wide range of scientific research applications, including:
Chemistry: Used as a fluorescent probe in various analytical techniques, such as fluorescence spectroscopy and chromatography.
Biology: Employed in the study of cellular processes, including cell imaging and tracking, due to its strong fluorescence.
Medicine: Investigated for its potential therapeutic properties, including anti-inflammatory, anticoagulant, and anticancer activities.
Industry: Utilized in the production of optical brighteners, laser dyes, and as a component in light-emitting diodes (LEDs).
Comparison with Similar Compounds
Similar Compounds
Coumarin: The parent compound, known for its pleasant aroma and use in fragrances and flavorings.
Warfarin: A well-known anticoagulant derived from coumarin, used to prevent blood clots.
Esculin: A coumarin derivative used in the treatment of hemorrhoids and as a diagnostic tool in microbiology.
Uniqueness of Coumarin 30
This compound stands out due to its superior photophysical properties, making it highly effective as a fluorescent dye. Its ability to form stable complexes with various metal ions and its versatility in chemical modifications further enhance its applicability in scientific research and industrial applications.
Properties
IUPAC Name |
7-(diethylamino)-3-(1-methylbenzimidazol-2-yl)chromen-2-one | |
---|---|---|
Source | PubChem | |
URL | https://pubchem.ncbi.nlm.nih.gov | |
Description | Data deposited in or computed by PubChem | |
InChI |
InChI=1S/C21H21N3O2/c1-4-24(5-2)15-11-10-14-12-16(21(25)26-19(14)13-15)20-22-17-8-6-7-9-18(17)23(20)3/h6-13H,4-5H2,1-3H3 | |
Source | PubChem | |
URL | https://pubchem.ncbi.nlm.nih.gov | |
Description | Data deposited in or computed by PubChem | |
InChI Key |
KZFUMWVJJNDGAU-UHFFFAOYSA-N | |
Source | PubChem | |
URL | https://pubchem.ncbi.nlm.nih.gov | |
Description | Data deposited in or computed by PubChem | |
Canonical SMILES |
CCN(CC)C1=CC2=C(C=C1)C=C(C(=O)O2)C3=NC4=CC=CC=C4N3C | |
Source | PubChem | |
URL | https://pubchem.ncbi.nlm.nih.gov | |
Description | Data deposited in or computed by PubChem | |
Molecular Formula |
C21H21N3O2 | |
Source | PubChem | |
URL | https://pubchem.ncbi.nlm.nih.gov | |
Description | Data deposited in or computed by PubChem | |
DSSTOX Substance ID |
DTXSID6068272 | |
Record name | 2H-1-Benzopyran-2-one, 7-(diethylamino)-3-(1-methyl-1H-benzimidazol-2-yl)- | |
Source | EPA DSSTox | |
URL | https://comptox.epa.gov/dashboard/DTXSID6068272 | |
Description | DSSTox provides a high quality public chemistry resource for supporting improved predictive toxicology. | |
Molecular Weight |
347.4 g/mol | |
Source | PubChem | |
URL | https://pubchem.ncbi.nlm.nih.gov | |
Description | Data deposited in or computed by PubChem | |
Physical Description |
Solid; [MSDSonline] | |
Record name | Coumarin 30 | |
Source | Haz-Map, Information on Hazardous Chemicals and Occupational Diseases | |
URL | https://haz-map.com/Agents/2184 | |
Description | Haz-Map® is an occupational health database designed for health and safety professionals and for consumers seeking information about the adverse effects of workplace exposures to chemical and biological agents. | |
Explanation | Copyright (c) 2022 Haz-Map(R). All rights reserved. Unless otherwise indicated, all materials from Haz-Map are copyrighted by Haz-Map(R). No part of these materials, either text or image may be used for any purpose other than for personal use. Therefore, reproduction, modification, storage in a retrieval system or retransmission, in any form or by any means, electronic, mechanical or otherwise, for reasons other than personal use, is strictly prohibited without prior written permission. | |
CAS No. |
41044-12-6 | |
Record name | 7-(Diethylamino)-3-(1-methyl-2-benzimidazolyl)coumarin | |
Source | CAS Common Chemistry | |
URL | https://commonchemistry.cas.org/detail?cas_rn=41044-12-6 | |
Description | CAS Common Chemistry is an open community resource for accessing chemical information. Nearly 500,000 chemical substances from CAS REGISTRY cover areas of community interest, including common and frequently regulated chemicals, and those relevant to high school and undergraduate chemistry classes. This chemical information, curated by our expert scientists, is provided in alignment with our mission as a division of the American Chemical Society. | |
Explanation | The data from CAS Common Chemistry is provided under a CC-BY-NC 4.0 license, unless otherwise stated. | |
Record name | Coumarin 30 | |
Source | ChemIDplus | |
URL | https://pubchem.ncbi.nlm.nih.gov/substance/?source=chemidplus&sourceid=0041044126 | |
Description | ChemIDplus is a free, web search system that provides access to the structure and nomenclature authority files used for the identification of chemical substances cited in National Library of Medicine (NLM) databases, including the TOXNET system. | |
Record name | Coumarin 30 | |
Source | DTP/NCI | |
URL | https://dtp.cancer.gov/dtpstandard/servlet/dwindex?searchtype=NSC&outputformat=html&searchlist=290433 | |
Description | The NCI Development Therapeutics Program (DTP) provides services and resources to the academic and private-sector research communities worldwide to facilitate the discovery and development of new cancer therapeutic agents. | |
Explanation | Unless otherwise indicated, all text within NCI products is free of copyright and may be reused without our permission. Credit the National Cancer Institute as the source. | |
Record name | 2H-1-Benzopyran-2-one, 7-(diethylamino)-3-(1-methyl-1H-benzimidazol-2-yl)- | |
Source | EPA Chemicals under the TSCA | |
URL | https://www.epa.gov/chemicals-under-tsca | |
Description | EPA Chemicals under the Toxic Substances Control Act (TSCA) collection contains information on chemicals and their regulations under TSCA, including non-confidential content from the TSCA Chemical Substance Inventory and Chemical Data Reporting. | |
Record name | 2H-1-Benzopyran-2-one, 7-(diethylamino)-3-(1-methyl-1H-benzimidazol-2-yl)- | |
Source | EPA DSSTox | |
URL | https://comptox.epa.gov/dashboard/DTXSID6068272 | |
Description | DSSTox provides a high quality public chemistry resource for supporting improved predictive toxicology. | |
Record name | 7-(diethylamino)-3-(1-methyl-1H-benzimidazol-2-yl)-2-benzopyrone | |
Source | European Chemicals Agency (ECHA) | |
URL | https://echa.europa.eu/substance-information/-/substanceinfo/100.050.152 | |
Description | The European Chemicals Agency (ECHA) is an agency of the European Union which is the driving force among regulatory authorities in implementing the EU's groundbreaking chemicals legislation for the benefit of human health and the environment as well as for innovation and competitiveness. | |
Explanation | Use of the information, documents and data from the ECHA website is subject to the terms and conditions of this Legal Notice, and subject to other binding limitations provided for under applicable law, the information, documents and data made available on the ECHA website may be reproduced, distributed and/or used, totally or in part, for non-commercial purposes provided that ECHA is acknowledged as the source: "Source: European Chemicals Agency, http://echa.europa.eu/". Such acknowledgement must be included in each copy of the material. ECHA permits and encourages organisations and individuals to create links to the ECHA website under the following cumulative conditions: Links can only be made to webpages that provide a link to the Legal Notice page. | |
Record name | Coumarin 30 | |
Source | FDA Global Substance Registration System (GSRS) | |
URL | https://gsrs.ncats.nih.gov/ginas/app/beta/substances/CT7C6AR5DD | |
Description | The FDA Global Substance Registration System (GSRS) enables the efficient and accurate exchange of information on what substances are in regulated products. Instead of relying on names, which vary across regulatory domains, countries, and regions, the GSRS knowledge base makes it possible for substances to be defined by standardized, scientific descriptions. | |
Explanation | Unless otherwise noted, the contents of the FDA website (www.fda.gov), both text and graphics, are not copyrighted. They are in the public domain and may be republished, reprinted and otherwise used freely by anyone without the need to obtain permission from FDA. Credit to the U.S. Food and Drug Administration as the source is appreciated but not required. | |
Retrosynthesis Analysis
AI-Powered Synthesis Planning: Our tool employs the Template_relevance Pistachio, Template_relevance Bkms_metabolic, Template_relevance Pistachio_ringbreaker, Template_relevance Reaxys, Template_relevance Reaxys_biocatalysis model, leveraging a vast database of chemical reactions to predict feasible synthetic routes.
One-Step Synthesis Focus: Specifically designed for one-step synthesis, it provides concise and direct routes for your target compounds, streamlining the synthesis process.
Accurate Predictions: Utilizing the extensive PISTACHIO, BKMS_METABOLIC, PISTACHIO_RINGBREAKER, REAXYS, REAXYS_BIOCATALYSIS database, our tool offers high-accuracy predictions, reflecting the latest in chemical research and data.
Strategy Settings
Precursor scoring | Relevance Heuristic |
---|---|
Min. plausibility | 0.01 |
Model | Template_relevance |
Template Set | Pistachio/Bkms_metabolic/Pistachio_ringbreaker/Reaxys/Reaxys_biocatalysis |
Top-N result to add to graph | 6 |
Feasible Synthetic Routes
Q1: What is the peak absorption wavelength of Coumarin 30?
A1: The peak absorption wavelength of this compound is typically around 410 nm. []
Q2: How does doping with Fullerene (C60) affect the photoconductive properties of this compound films?
A2: Doping this compound films with C60 significantly improves their photoconductive properties. This is due to the enhanced dissociation of electron-hole pairs at the this compound/C60 interface, leading to higher external quantum efficiency. []
Q3: Can this compound be used in two-photon fluorescence (TPF) applications?
A3: Yes, this compound exhibits two-photon fluorescence. Research shows that optimizing the excitation pulse shape using femtosecond laser pulses can significantly enhance its TPF signal. This has potential applications in fields like bio-imaging. [, ]
Q4: How does this compound contribute to the performance of organic solar cells?
A4: While not a primary absorber, this compound can be incorporated as a third component in ternary organic solar cells. Research has shown that specific Coumarin derivatives like Coumarin 7, when added to a PTB7-Th:ITIC system, can enhance power conversion efficiency by facilitating charge transfer and optimizing the morphology of the photoactive layer. []
Q5: What causes the change in the gain spectrum of this compound when used in a dye mixture with Rhodamine 6G?
A5: The shift in the gain spectrum peak in this compound and Rhodamine 6G mixtures is attributed to energy transfer between the dyes. This energy transfer primarily occurs through a radiative mechanism, leading to a change in the effective fluorescence lifetime of this compound. []
Q6: Has this compound been used in laser applications?
A6: Yes, this compound has been successfully utilized in various laser applications. For instance, it has been employed in a dye laser amplifier where its output polarization is influenced by both the Kerr effect of the solvent and the anisotropic orientation of excited this compound molecules. [] It has also shown lasing capabilities in optically pumped, vacuum-deposited amorphous thin films, exhibiting low lasing thresholds and high differential quantum efficiencies. []
Q7: Can this compound be used as a sensitizer in photoconducting polymers?
A7: Research indicates that this compound can act as a sensitizer in soluble photoconducting polyimides (PIs). The efficiency of this sensitization, as well as the fluorescence quenching observed, is dependent on the type and strength of electron donor-acceptor interactions within these polymers. []
Q8: How does the aggregation of this compound in water affect its properties?
A8: this compound tends to self-aggregate in water, forming H-type aggregates. This aggregation leads to changes in the absorption and fluorescence properties of the dye. Studies have shown that β-cyclodextrin can encapsulate this compound monomers, effectively modulating the aggregation process. []
Q9: How does ionic strength affect the aggregation of this compound in an aqueous environment?
A9: Increasing the ionic strength of an aqueous solution by adding soluble salts accelerates the aggregation of this compound. This effect is primarily attributed to the “primary kinetic salt effect” rather than the “salting-out effect.” []
Q10: What is the impact of solvent polarity on the photophysical properties of this compound?
A10: this compound displays solvent-dependent photophysical properties. Studies have shown that in high-polarity protic solvents, excited this compound undergoes a nonradiative deexcitation pathway involving a twisted intramolecular charge transfer (TICT) state. This contrasts with its behavior in aprotic solvents, where TICT state formation is less prominent. [, ]
Q11: How does the rotational diffusion of this compound compare in different solvents?
A11: Research indicates that the rotational diffusion of this compound is influenced by the solvent environment. Studies comparing its behavior in alkane and alcohol solvents demonstrate that this compound rotates faster in alkanes than in alcohols. This difference is attributed to variations in the friction experienced by the dye molecule in each solvent type. [, , ]
Q12: Can you elaborate on the impact of hydrogen bonding on the photophysical properties of this compound?
A12: Hydrogen bonding plays a significant role in the photophysical behavior of this compound. In protic solvents, intermolecular hydrogen bonding between the dye and solvent molecules influences its fluorescence quantum yield and lifetime. [, ] Furthermore, the presence of an intramolecular hydrogen bond within the Coumarin 7 molecule, a close derivative of this compound, has been shown to affect the formation of its TICT state. []
Q13: How does temperature affect the fluorescence lifetime of this compound?
A13: Temperature variations can impact the fluorescence lifetime of this compound. For example, in ethanol solution, the fluorescence lifetime of this compound, when excited at 400 nm, has been measured to be (1.9 ± 0.3) ns using femtosecond laser pulses. [] These measurements, typically performed using techniques like time-correlated single photon counting or femtosecond pump-probe, provide insights into the excited state dynamics of the dye.
Q14: How can this compound be utilized in the fabrication of metal nanoparticle-polymer nanocomposites?
A14: this compound has demonstrated utility in the fabrication of metal nanoparticle–polymer nanocomposites using two-photon polymerization (2PP). Its role as a photosensitive dye is particularly useful in the in situ formation of silver nanoparticles (AgNPs) within the polymer matrix. The addition of this compound improves both the reduction of silver ions and the overall distribution of AgNPs within the composite material. []
Q15: Can this compound be used to create optical phantoms that mimic tissue properties?
A15: Yes, this compound is a suitable fluorophore for creating optical phantoms that simulate the optical properties of biological tissues. These phantoms are often composed of an agarose-based matrix incorporating scatterers like silicon dioxide and Intralipid, absorbers like ink and blood, and fluorophores such as this compound to mimic tissue autofluorescence. Such phantoms find applications in research areas like photodynamic therapy development and fluorescence spectroscopy. [, , ]
Q16: How is this compound used to study interfaces in polymer nanocomposites?
A16: this compound plays a crucial role in a technique that utilizes Förster resonance energy transfer (FRET) to analyze interfaces within polymer nanocomposites. In a model system, nanofibrillated cellulose labeled with a fluorescent dye is dispersed into polyethylene doped with this compound. By monitoring the FRET between the two dyes, researchers can assess the extent of interface formation and the homogeneity of the composite material. This method provides valuable insights into the nanoscale structure of such composites and how processing affects their properties. []
Disclaimer and Information on In-Vitro Research Products
Please be aware that all articles and product information presented on BenchChem are intended solely for informational purposes. The products available for purchase on BenchChem are specifically designed for in-vitro studies, which are conducted outside of living organisms. In-vitro studies, derived from the Latin term "in glass," involve experiments performed in controlled laboratory settings using cells or tissues. It is important to note that these products are not categorized as medicines or drugs, and they have not received approval from the FDA for the prevention, treatment, or cure of any medical condition, ailment, or disease. We must emphasize that any form of bodily introduction of these products into humans or animals is strictly prohibited by law. It is essential to adhere to these guidelines to ensure compliance with legal and ethical standards in research and experimentation.