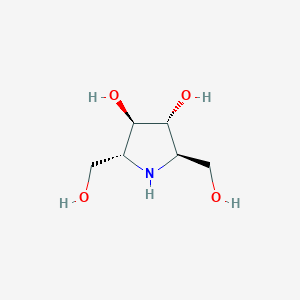
2,5-Dideoxy-2,5-imino-D-mannitol
Overview
Description
2,5-Dideoxy-2,5-imino-D-mannitol is a naturally occurring iminosugar that has been isolated from various plant sources. It is known for its potent inhibitory activity against glycosidases, enzymes that play a crucial role in the hydrolysis of glycosidic bonds in carbohydrates.
Mechanism of Action
Target of Action
2,5-Dideoxy-2,5-imino-D-mannitol (DMDP) primarily targets α-glucosidase (α-GLU) . α-GLU is an enzyme that hydrolyzes starch and disaccharides by targeting terminal, non-reducing (1→4)-linked α-D-glucose residues to produce α-glucose . It is substrate selective .
Mode of Action
DMDP acts as a glycosidase inhibitor . Glycosidases are enzymes responsible for the cleavage of glycosidic bonds. DMDP, being a powerful inhibitor of these enzymes, associates with acidic amino acids at the active site . This interaction inhibits the normal function of the enzyme, thereby altering the biochemical processes that rely on the enzyme’s activity.
Biochemical Pathways
The inhibition of α-GLU by DMDP affects the carbohydrate processing pathways . These pathways are responsible for glycoprotein processing and the gastrointestinal breakdown of dietary carbohydrates . By inhibiting α-GLU, DMDP disrupts these pathways, leading to an accumulation of undigested carbohydrates and affecting energy metabolism.
Result of Action
The primary result of DMDP’s action is the inhibition of α-GLU , leading to the disruption of carbohydrate processing pathways . This can have various downstream effects depending on the specific biological context. For instance, DMDP has been shown to have antiviral properties by inhibiting viral DNA polymerase, thereby preventing viral replication .
Preparation Methods
Synthetic Routes and Reaction Conditions
Several synthetic approaches have been developed for the preparation of 2,5-Dideoxy-2,5-imino-D-mannitol. One common method involves the stereospecific synthesis from protected sugar hemiacetals. This process typically includes the formation of N-tert-butanesulfinyl glycosylamines, followed by reduction and deprotection steps . Another approach involves the use of pyrrolidine derivatives, which are powerful inhibitors of glycohydrolases .
Industrial Production Methods
Industrial production methods for this compound are not well-documented in the literature. the synthetic routes mentioned above can be adapted for large-scale production with appropriate optimization of reaction conditions and purification techniques.
Chemical Reactions Analysis
Types of Reactions
2,5-Dideoxy-2,5-imino-D-mannitol undergoes various chemical reactions, including oxidation, reduction, and substitution. These reactions are often facilitated by the presence of hydroxyl and amino groups in the molecule.
Common Reagents and Conditions
Oxidation: Common oxidizing agents such as potassium permanganate and hydrogen peroxide can be used to oxidize this compound.
Reduction: Reducing agents like sodium borohydride and lithium aluminum hydride are employed to reduce the compound.
Substitution: Nucleophilic substitution reactions can be carried out using reagents like alkyl halides and acyl chlorides.
Major Products
Scientific Research Applications
2,5-Dideoxy-2,5-imino-D-mannitol has been extensively studied for its applications in various scientific fields:
Chemistry: It is used as a glycosidase inhibitor in the study of carbohydrate metabolism and enzyme mechanisms.
Biology: The compound is employed in glycobiology research to investigate the role of glycosidases in cellular processes.
Medicine: Due to its inhibitory activity against glycosidases, this compound has potential therapeutic applications in the treatment of diseases such as diabetes and viral infections.
Industry: The compound is used in the development of novel pharmaceuticals and agrochemicals.
Comparison with Similar Compounds
2,5-Dideoxy-2,5-imino-D-mannitol is unique among iminosugars due to its specific inhibitory activity against glycosidases. Similar compounds include:
2,5-Dideoxy-2,5-imino-D-glucitol: Another iminosugar with similar inhibitory properties but different structural features.
2,5-Dideoxy-2,5-imino-D-glycero-D-manno-heptitol: A heptitol derivative with glycosidase inhibitory activity.
1-Deoxynojirimycin: A well-known iminosugar with potent glycosidase inhibitory activity
These compounds share similar mechanisms of action but differ in their structural configurations and specific enzyme targets.
Properties
IUPAC Name |
2,5-bis(hydroxymethyl)pyrrolidine-3,4-diol | |
---|---|---|
Details | Computed by LexiChem 2.6.6 (PubChem release 2019.06.18) | |
Source | PubChem | |
URL | https://pubchem.ncbi.nlm.nih.gov | |
Description | Data deposited in or computed by PubChem | |
InChI |
InChI=1S/C6H13NO4/c8-1-3-5(10)6(11)4(2-9)7-3/h3-11H,1-2H2 | |
Details | Computed by InChI 1.0.5 (PubChem release 2019.06.18) | |
Source | PubChem | |
URL | https://pubchem.ncbi.nlm.nih.gov | |
Description | Data deposited in or computed by PubChem | |
InChI Key |
PFYHYHZGDNWFIF-UHFFFAOYSA-N | |
Details | Computed by InChI 1.0.5 (PubChem release 2019.06.18) | |
Source | PubChem | |
URL | https://pubchem.ncbi.nlm.nih.gov | |
Description | Data deposited in or computed by PubChem | |
Canonical SMILES |
C(C1C(C(C(N1)CO)O)O)O | |
Details | Computed by OEChem 2.1.5 (PubChem release 2019.06.18) | |
Source | PubChem | |
URL | https://pubchem.ncbi.nlm.nih.gov | |
Description | Data deposited in or computed by PubChem | |
Molecular Formula |
C6H13NO4 | |
Details | Computed by PubChem 2.1 (PubChem release 2019.06.18) | |
Source | PubChem | |
URL | https://pubchem.ncbi.nlm.nih.gov | |
Description | Data deposited in or computed by PubChem | |
Molecular Weight |
163.17 g/mol | |
Details | Computed by PubChem 2.1 (PubChem release 2021.05.07) | |
Source | PubChem | |
URL | https://pubchem.ncbi.nlm.nih.gov | |
Description | Data deposited in or computed by PubChem | |
Retrosynthesis Analysis
AI-Powered Synthesis Planning: Our tool employs the Template_relevance Pistachio, Template_relevance Bkms_metabolic, Template_relevance Pistachio_ringbreaker, Template_relevance Reaxys, Template_relevance Reaxys_biocatalysis model, leveraging a vast database of chemical reactions to predict feasible synthetic routes.
One-Step Synthesis Focus: Specifically designed for one-step synthesis, it provides concise and direct routes for your target compounds, streamlining the synthesis process.
Accurate Predictions: Utilizing the extensive PISTACHIO, BKMS_METABOLIC, PISTACHIO_RINGBREAKER, REAXYS, REAXYS_BIOCATALYSIS database, our tool offers high-accuracy predictions, reflecting the latest in chemical research and data.
Strategy Settings
Precursor scoring | Relevance Heuristic |
---|---|
Min. plausibility | 0.01 |
Model | Template_relevance |
Template Set | Pistachio/Bkms_metabolic/Pistachio_ringbreaker/Reaxys/Reaxys_biocatalysis |
Top-N result to add to graph | 6 |
Feasible Synthetic Routes
A: DMDP primarily functions as a glycosidase inhibitor. [, , , , ] These enzymes catalyze the hydrolysis of glycosidic bonds in carbohydrates. DMDP mimics the structure of the natural sugar substrate and binds to the active site of glycosidases, thereby blocking the enzyme's action. [, , ] This inhibition can disrupt various cellular processes that rely on glycosidases, such as carbohydrate metabolism and glycoprotein processing. [, , ]
A: DMDP demonstrates inhibitory activity against various glycosidases, including β-glucosidases, α-glucosidases, β-galactosidases, and α-L-fucosidases. [, , , , , , ] Notably, its inhibitory profile can vary depending on the specific enzyme and its source. [, , , ] For example, while DMDP effectively inhibits processing α-glucosidase I, it has minimal impact on processing α-glucosidase II. []
ANone: DMDP has the molecular formula C6H13NO4 and a molecular weight of 163.17 g/mol.
ANone: While the provided papers don't delve into specific spectroscopic details, researchers typically characterize DMDP using techniques like Nuclear Magnetic Resonance (NMR) spectroscopy and Mass Spectrometry (MS). These methods help to confirm the structure, determine purity, and analyze the compound's behavior in various environments.
ANone: The provided research papers primarily focus on the biological activity and synthesis of DMDP. Information regarding its material compatibility and stability under various conditions isn't directly addressed. Further research is needed to explore these aspects.
ANone: DMDP is not generally recognized for having catalytic properties. Its primary mode of action revolves around inhibiting enzymes rather than catalyzing reactions.
ANone: While the provided research papers don't provide detailed information on computational studies concerning DMDP, these techniques are widely used in drug discovery. Researchers could employ molecular docking simulations to understand how DMDP interacts with the active site of various glycosidases. This information can guide the design of more potent and selective inhibitors.
A: Modifications to the DMDP structure can significantly influence its inhibitory potency and selectivity towards different glycosidases. [, , , , ] For instance, N-alkylation of DMDP generally reduces its inhibitory activity against various enzymes, except for α-glucosidase I. [] This suggests that the free nitrogen on the pyrrolidine ring plays a crucial role in binding to most target enzymes. [] Introducing different substituents at the C-1 position of DMDP can either enhance or diminish its inhibitory activity depending on the nature of the modification. [, ]
ANone: The provided research primarily focuses on DMDP's synthesis and biological activity. Data regarding its stability under various conditions and formulation strategies to improve its bioavailability is limited in the provided context.
ANone: The research papers provided primarily focus on the scientific aspects of DMDP, without delving into specific SHE (Safety, Health, and Environment) regulations. As with all chemical compounds, handling DMDP requires adherence to standard laboratory safety procedures and appropriate waste disposal protocols.
ANone: The provided research papers mainly focus on the in vitro activity and structural aspects of DMDP, with limited information on its PK/PD properties. Further studies are needed to determine its absorption, distribution, metabolism, and excretion (ADME) profile, and understand its in vivo activity and efficacy in different animal models.
A: DMDP has demonstrated antihyperglycemic effects in streptozotocin-induced diabetic mice. [] This effect might be partially attributed to its ability to increase plasma insulin levels and enhance glucose-induced insulin release from the pancreas. [] Further research is needed to fully elucidate the mechanism behind its antihyperglycemic action.
ANone: The provided research papers do not specifically address the development of resistance to DMDP. Understanding potential resistance mechanisms and cross-resistance with other glycosidase inhibitors requires further investigation.
ANone: While these aspects are crucial for a comprehensive understanding of DMDP, the provided research papers primarily focus on its synthesis, structure-activity relationship, and inhibitory activity against glycosidases. Further research is necessary to explore these additional aspects thoroughly.
A: DMDP was first isolated from the tropical plant Angylocalyx Pynaertii. [] Since its discovery, research on DMDP has expanded to explore its synthesis, biological activity, and potential therapeutic applications. [] The development of various synthetic routes to DMDP, including those utilizing readily available starting materials like D-mannitol and L-xylose, marks a significant achievement in the field. [, ]
A: Research on DMDP bridges several disciplines, including organic chemistry, biochemistry, and pharmacology. [] Its role as a glycosidase inhibitor makes it a valuable tool in glycobiology research, aiding in the study of carbohydrate-processing enzymes and their functions. [, ] Furthermore, its potential therapeutic applications, particularly in the context of diabetes, highlight the importance of collaborative efforts between chemists, biologists, and clinicians to further explore its therapeutic potential. [, ]
Disclaimer and Information on In-Vitro Research Products
Please be aware that all articles and product information presented on BenchChem are intended solely for informational purposes. The products available for purchase on BenchChem are specifically designed for in-vitro studies, which are conducted outside of living organisms. In-vitro studies, derived from the Latin term "in glass," involve experiments performed in controlled laboratory settings using cells or tissues. It is important to note that these products are not categorized as medicines or drugs, and they have not received approval from the FDA for the prevention, treatment, or cure of any medical condition, ailment, or disease. We must emphasize that any form of bodily introduction of these products into humans or animals is strictly prohibited by law. It is essential to adhere to these guidelines to ensure compliance with legal and ethical standards in research and experimentation.