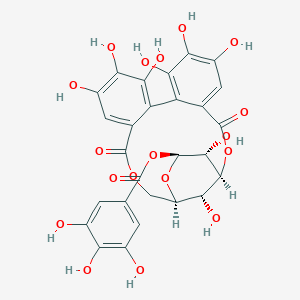
Corilagin
Overview
Description
Corilagin is a type of ellagitannin, a class of hydrolyzable tannins, which are polyphenolic compounds. It was first isolated in 1951 from the extract of Dividivi (Caesalpinia coriaria) and has since been found in various plants such as Alchornea glandulosa and the leaves of Punica granatum (pomegranate) . This compound is known for its broad-spectrum biological and therapeutic activities, including antioxidant, anti-inflammatory, hepatoprotective, and antitumor actions .
Mechanism of Action
Target of Action
Corilagin, a gallotannin identified in several plants, has been found to interact with various targets in the body. It has been shown to bind with plasma serum proteins like human serum albumin (HSA) and α-1-acid glycoprotein (AGP) under physiological conditions . It also targets the NOD-like receptor pyrin domain containing 3 (NLRP3) inflammasome, which significantly influences atherosclerotic progression . Furthermore, it has been found to interact with key proteins in the MAPK signaling pathway .
Mode of Action
This compound’s interaction with its targets leads to various changes in the body. For instance, it has been found to cause an increase in the inhibition growth of inflamed macrophages in a concentration-dependent manner . It also inhibits the activation of both the canonical Smad and non-canonical extracellular-signal-regulated kinase/Akt (protein kinase B) pathways . Furthermore, it effectively inhibits NLRP3 inflammasome activation, consequently diminishing inflammation, macrophage polarization, and pyroptosis .
Biochemical Pathways
This compound affects various biochemical pathways. It has been found to downregulate the LOX-1/MyD88/NF-κB pathway, which is associated with antiatherosclerotic effects . It also modulates the TOLL-like receptor signaling pathway and the MAPK signaling pathway .
Result of Action
The molecular and cellular effects of this compound’s action are diverse. It has been shown to prompt cell cycle arrest at the G2/M phase and augment apoptosis . It also induces apoptosis and autophagic cell death depending on the production of intracellular reactive oxygen species in breast cancer cell lines . Furthermore, it suppresses cholangiocarcinoma growth and downregulates the expression of Notch1 and mammalian target of rapamycin .
Action Environment
The action, efficacy, and stability of this compound can be influenced by various environmental factors. For instance, the presence of lipopolysaccharides and oxidized low-density lipoprotein can affect the anti-inflammatory effect of this compound . .
Biochemical Analysis
Biochemical Properties
Corilagin plays a crucial role in various biochemical reactions. It interacts with several enzymes, proteins, and other biomolecules, influencing their activity and function. For instance, this compound has been shown to inhibit the activity of nuclear factor-kappa B (NF-κB), a protein complex that plays a key role in regulating the immune response to infection . Additionally, this compound interacts with myeloid differentiation factor 88 (MyD88) and lectin-like oxidized low-density lipoprotein receptor-1 (LOX-1), modulating their expression and activity . These interactions contribute to this compound’s anti-inflammatory and antiatherosclerotic effects.
Cellular Effects
This compound exerts various effects on different types of cells and cellular processes. It has been shown to influence cell function by modulating cell signaling pathways, gene expression, and cellular metabolism. For example, this compound inhibits the proliferation and migration of vascular smooth muscle cells (VSMCs) induced by oxidized low-density lipoprotein (ox-LDL), thereby reducing the formation of foam cells and intimal thickening in the thoracic aorta . Additionally, this compound induces apoptosis and autophagic cell death in cancer cells by generating intracellular reactive oxygen species and blocking the activation of both the canonical Smad and non-canonical extracellular-signal-regulated kinase/Akt pathways .
Molecular Mechanism
The molecular mechanism of this compound involves several binding interactions with biomolecules, enzyme inhibition or activation, and changes in gene expression. This compound binds to and inhibits the activity of NF-κB, reducing the expression of pro-inflammatory cytokines such as tumor necrosis factor α (TNF-α) and monocyte chemoattractant protein-1 (MCP-1) . It also downregulates the expression of LOX-1 and MyD88, further contributing to its anti-inflammatory effects . In cancer cells, this compound induces apoptosis by altering the expression of procaspase-3, procaspase-8, procaspase-9, poly (ADP ribose) polymerase, and Bcl-2 Bax .
Temporal Effects in Laboratory Settings
In laboratory settings, the effects of this compound have been observed to change over time. This compound’s stability and degradation play a significant role in its long-term effects on cellular function. Studies have shown that this compound maintains its stability and efficacy over extended periods, allowing for sustained anti-inflammatory and antiatherosclerotic effects
Dosage Effects in Animal Models
The effects of this compound vary with different dosages in animal models. At lower doses, this compound has been shown to exert beneficial effects, such as reducing serum lipid levels and inhibiting the proliferation of VSMCs . At higher doses, this compound may exhibit toxic or adverse effects, highlighting the importance of determining the optimal dosage for therapeutic use. Studies have also reported threshold effects, where the efficacy of this compound plateaus beyond a certain dosage .
Metabolic Pathways
This compound is involved in several metabolic pathways, interacting with various enzymes and cofactors. It influences metabolic flux and metabolite levels, contributing to its therapeutic effects. For instance, this compound has been shown to modulate lipid metabolism by decreasing lipid deposition and maintaining the homeostasis of lipid metabolism . Additionally, this compound’s interaction with enzymes involved in oxidative stress pathways further enhances its antioxidant properties .
Transport and Distribution
This compound is transported and distributed within cells and tissues through specific transporters and binding proteins. These interactions influence its localization and accumulation, affecting its overall efficacy. Studies have shown that this compound is efficiently taken up by cells and distributed to various tissues, allowing for its widespread therapeutic effects . The transport and distribution of this compound are crucial for its ability to exert its biological activities.
Subcellular Localization
The subcellular localization of this compound plays a significant role in its activity and function. This compound is directed to specific compartments or organelles through targeting signals and post-translational modifications. For example, this compound has been shown to localize in the cytoplasm and nucleus, where it interacts with key signaling molecules and transcription factors . This subcellular localization is essential for this compound’s ability to modulate gene expression and cellular processes.
Preparation Methods
Synthetic Routes and Reaction Conditions
Corilagin can be prepared using a green extraction and purification process from plants like Phyllanthus. The process involves mixing the plant material with an aqueous ionic liquid, such as 0.4 M [BMIm]Br, at a liquid-solid ratio of 10:1 and dispersing the mixture by ultrasonication at 50°C for 15 minutes. The mixture is then subjected to macroporous resin D101 and preparative high-performance liquid chromatography (prep-HPLC) for separation, followed by water precipitation to achieve high purity .
Industrial Production Methods
An industrial method for extracting and purifying this compound involves subjecting common leafflower herb to absorption by macroporous resin, desorption, and extraction with n-butyl alcohol. The concentrated extraction solution is further purified using supercritical carbon dioxide fluid and gel column chromatography, followed by recrystallization to obtain this compound .
Chemical Reactions Analysis
Types of Reactions
Corilagin undergoes various chemical reactions, including oxidation, reduction, and substitution. It has shown inhibitory activity against the growth of numerous cancer cells by prompting cell cycle arrest at the G2/M phase and augmented apoptosis .
Common Reagents and Conditions
Common reagents used in the reactions involving this compound include reactive oxygen species (ROS) scavengers and various solvents like methanol, ethanol, and acetone. The conditions often involve specific temperatures and pH levels to optimize the reactions .
Major Products Formed
The major products formed from the reactions involving this compound include ellagic acid and gallic acid, which exhibit differences in bioavailability and half-life compared to the parent compound .
Scientific Research Applications
Comparison with Similar Compounds
Corilagin is unique among ellagitannins due to its specific molecular structure and broad-spectrum biological activities. Similar compounds include:
Ellagic Acid: Another ellagitannin with antioxidant and anticancer properties.
Gallic Acid: A hydrolytic product of this compound with differences in bioavailability and half-life.
Punicalagin: Found in pomegranate, known for its antioxidant and anti-inflammatory effects.
Properties
IUPAC Name |
[(1S,19R,21S,22R,23R)-6,7,8,11,12,13,22,23-octahydroxy-3,16-dioxo-2,17,20-trioxatetracyclo[17.3.1.04,9.010,15]tricosa-4,6,8,10,12,14-hexaen-21-yl] 3,4,5-trihydroxybenzoate | |
---|---|---|
Source | PubChem | |
URL | https://pubchem.ncbi.nlm.nih.gov | |
Description | Data deposited in or computed by PubChem | |
InChI |
InChI=1S/C27H22O18/c28-9-1-6(2-10(29)16(9)32)24(39)45-27-22(38)23-19(35)13(43-27)5-42-25(40)7-3-11(30)17(33)20(36)14(7)15-8(26(41)44-23)4-12(31)18(34)21(15)37/h1-4,13,19,22-23,27-38H,5H2/t13-,19-,22-,23+,27+/m1/s1 | |
Source | PubChem | |
URL | https://pubchem.ncbi.nlm.nih.gov | |
Description | Data deposited in or computed by PubChem | |
InChI Key |
TUSDEZXZIZRFGC-XIGLUPEJSA-N | |
Source | PubChem | |
URL | https://pubchem.ncbi.nlm.nih.gov | |
Description | Data deposited in or computed by PubChem | |
Canonical SMILES |
C1C2C(C(C(C(O2)OC(=O)C3=CC(=C(C(=C3)O)O)O)O)OC(=O)C4=CC(=C(C(=C4C5=C(C(=C(C=C5C(=O)O1)O)O)O)O)O)O)O | |
Source | PubChem | |
URL | https://pubchem.ncbi.nlm.nih.gov | |
Description | Data deposited in or computed by PubChem | |
Isomeric SMILES |
C1[C@@H]2[C@H]([C@@H]([C@H]([C@@H](O2)OC(=O)C3=CC(=C(C(=C3)O)O)O)O)OC(=O)C4=CC(=C(C(=C4C5=C(C(=C(C=C5C(=O)O1)O)O)O)O)O)O)O | |
Source | PubChem | |
URL | https://pubchem.ncbi.nlm.nih.gov | |
Description | Data deposited in or computed by PubChem | |
Molecular Formula |
C27H22O18 | |
Source | PubChem | |
URL | https://pubchem.ncbi.nlm.nih.gov | |
Description | Data deposited in or computed by PubChem | |
DSSTOX Substance ID |
DTXSID301104707 | |
Record name | β-D-Glucopyranose, cyclic 3,6-(4,4′,5,5′,6,6′-hexahydroxy[1,1′-biphenyl]-2,2′-dicarboxylate) 1-(3,4,5-trihydroxybenzoate) | |
Source | EPA DSSTox | |
URL | https://comptox.epa.gov/dashboard/DTXSID301104707 | |
Description | DSSTox provides a high quality public chemistry resource for supporting improved predictive toxicology. | |
Molecular Weight |
634.5 g/mol | |
Source | PubChem | |
URL | https://pubchem.ncbi.nlm.nih.gov | |
Description | Data deposited in or computed by PubChem | |
CAS No. |
2088321-44-0, 23094-69-1 | |
Record name | β-D-Glucopyranose, cyclic 3,6-(4,4′,5,5′,6,6′-hexahydroxy[1,1′-biphenyl]-2,2′-dicarboxylate) 1-(3,4,5-trihydroxybenzoate) | |
Source | CAS Common Chemistry | |
URL | https://commonchemistry.cas.org/detail?cas_rn=2088321-44-0 | |
Description | CAS Common Chemistry is an open community resource for accessing chemical information. Nearly 500,000 chemical substances from CAS REGISTRY cover areas of community interest, including common and frequently regulated chemicals, and those relevant to high school and undergraduate chemistry classes. This chemical information, curated by our expert scientists, is provided in alignment with our mission as a division of the American Chemical Society. | |
Explanation | The data from CAS Common Chemistry is provided under a CC-BY-NC 4.0 license, unless otherwise stated. | |
Record name | β-D-Glucopyranose, cyclic 3,6-(4,4′,5,5′,6,6′-hexahydroxy[1,1′-biphenyl]-2,2′-dicarboxylate) 1-(3,4,5-trihydroxybenzoate) | |
Source | EPA DSSTox | |
URL | https://comptox.epa.gov/dashboard/DTXSID301104707 | |
Description | DSSTox provides a high quality public chemistry resource for supporting improved predictive toxicology. | |
Record name | Corilagin | |
Source | Human Metabolome Database (HMDB) | |
URL | http://www.hmdb.ca/metabolites/HMDB0031457 | |
Description | The Human Metabolome Database (HMDB) is a freely available electronic database containing detailed information about small molecule metabolites found in the human body. | |
Explanation | HMDB is offered to the public as a freely available resource. Use and re-distribution of the data, in whole or in part, for commercial purposes requires explicit permission of the authors and explicit acknowledgment of the source material (HMDB) and the original publication (see the HMDB citing page). We ask that users who download significant portions of the database cite the HMDB paper in any resulting publications. | |
Disclaimer and Information on In-Vitro Research Products
Please be aware that all articles and product information presented on BenchChem are intended solely for informational purposes. The products available for purchase on BenchChem are specifically designed for in-vitro studies, which are conducted outside of living organisms. In-vitro studies, derived from the Latin term "in glass," involve experiments performed in controlled laboratory settings using cells or tissues. It is important to note that these products are not categorized as medicines or drugs, and they have not received approval from the FDA for the prevention, treatment, or cure of any medical condition, ailment, or disease. We must emphasize that any form of bodily introduction of these products into humans or animals is strictly prohibited by law. It is essential to adhere to these guidelines to ensure compliance with legal and ethical standards in research and experimentation.