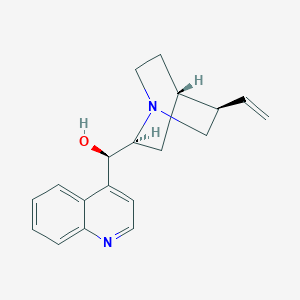
Cinchonidine
Overview
Description
Cinchonidine is an alkaloid found in Cinchona officinalis and Gongronema latifolium. It is used in asymmetric synthesis in organic chemistry . It belongs to the orthorhombic crystal system and P 212121 space group .
Synthesis Analysis
This compound has been used in the synthesis of novel molecules. For example, ten novel molecules have been designed with an amalgamation of this compound, carbohydrate moiety, and triazole ring by utilizing copper-catalyzed click reaction of this compound-derived azide and clickable glycosyl alkynes .
Molecular Structure Analysis
This compound has a molecular formula of C19H22N2O and a molecular weight of 294.4 g/mol . The structure of this compound includes three conformers that are substantially populated at room temperature .
Chemical Reactions Analysis
This compound and its stereoisomer cinchonine have long been used to direct chirality in the synthesis of asymmetric organic compounds . Dutch chemists have created chiral metals by crystallizing their salts in the presence of this compound .
Physical and Chemical Properties Analysis
This compound has a molar mass of 294.43 g/mol. It has a density of 1.2 g/mL, a melting point of 204 to 205 °C, and a boiling point of 464.5 °C. It is slightly soluble in water .
Scientific Research Applications
1. Antimalarial Activity
Cinchonidine has been studied for its antimalarial properties. It's one of the main alkaloids found in the Cinchona herb, used traditionally and in modern medicine for treating malaria (Gurung & De, 2017). Another study highlights novel cinchona alkaloid derivatives, including this compound, as potential antimalarial agents, emphasizing their interaction with malaria parasites (Rosmalena, Prasasty, & Hanafi, 2018).
2. Pharmacological Activities
This compound has been identified for its wide range of pharmacological activities, including potential anti-cancer, antioxidant, and anti-inflammatory effects. This variety of biological activities makes it a significant compound in pharmacognosy (Dubey & Singh, 2021).
3. Chemical Properties and Applications
The chemical properties of this compound, like its conformational behavior in different solvents, have been explored to understand its behavior in chemical reactions and its potential applications in catalysis and organic synthesis (Bürgi & Baiker, 1998). For example, this compound has been used as a chiral catalyst in asymmetric synthesis (Kojima, Suzuki, Watanabe, & Ohkata, 2006).
4. Enantioselective Catalysis
This compound has been tethered to porous silica, demonstrating its use as a solid chiral catalyst in enantioselective catalysis (Hong, Lee, & Zaera, 2011). This application is essential in producing pharmaceuticals and fine chemicals with specific optical properties.
5. Anticholinesterase Potency
This compound-based compounds have shown potential as selective inhibitors for enzymes like butyrylcholinesterase, which can have applications in treating neurodegenerative diseases (Bosak, Ramić, Šmidlehner, Hrenar, Primožič, & Kovarik, 2018).
Mechanism of Action
Target of Action
Cinchonidine, a major member of the Cinchona alkaloids, is primarily used as an antimalarial agent . Its primary targets are the malaria-causing Plasmodium parasites . These parasites are responsible for the degradation of hemoglobin in erythrocytes .
Mode of Action
It is generally assumed that this compound, like other cinchona alkaloids, prevents the polymerization of the toxic hematin (aquafe(iii)protoporphyrin ix) formed by the degradation of hemoglobin in erythrocytes to hemozoin (β-hematin) . This interaction inhibits the growth and multiplication of the Plasmodium parasites .
Biochemical Pathways
The biochemical pathways affected by this compound are related to the lifecycle of the Plasmodium parasites. By preventing the polymerization of toxic hematin, this compound disrupts the parasites’ ability to grow and multiply within red blood cells . This disruption affects the downstream effects of the parasites’ lifecycle, including their ability to cause the symptoms associated with malaria .
Pharmacokinetics
It has been reported that the mean plasma concentration for this compound is approximately 25 mg/L . This suggests that this compound has a significant bioavailability, which is crucial for its effectiveness as an antimalarial agent .
Result of Action
The molecular and cellular effects of this compound’s action primarily involve the inhibition of the growth and multiplication of Plasmodium parasites within red blood cells . This inhibition results in a decrease in the severity of malaria symptoms and can potentially save lives . Additionally, this compound has been reported to exhibit cell-protective effects against certain types of cell damage .
Action Environment
The action, efficacy, and stability of this compound can be influenced by various environmental factors. For instance, the natural environmental conditions such as temperature and humidity, as well as the type of soil, can have a substantial impact on the germination of Cinchona plants, which produce this compound . This suggests that the production and availability of this compound can be influenced by environmental conditions, which in turn can affect its action and efficacy .
Safety and Hazards
Future Directions
Biochemical Analysis
Biochemical Properties
Cinchonidine, like other Cinchona alkaloids, plays a significant role in biochemical reactions. It interacts with various enzymes, proteins, and other biomolecules . For instance, it has been found to exhibit cell-protective effects in indoxyl sulfate-stimulated human umbilical vein endothelial cells (HUVECs) .
Cellular Effects
This compound has been shown to have substantial effects on various types of cells and cellular processes. In HUVECs, it has been found to reverse indoxyl sulfate-induced cell death, cellular senescence, and impairment of tube formation .
Molecular Mechanism
This compound exerts its effects at the molecular level through various mechanisms. For instance, it has been found to downregulate the p53 signaling pathway in IS-treated HUVECs, promoting the degradation of p53 and the cytoplasmic-nuclear shuttling of MDM2 .
Properties
{ "Design of the Synthesis Pathway": "The synthesis pathway for Cinchonidine involves several steps of chemical reactions starting from simple starting materials.", "Starting Materials": [ "Quinoline", "Ethyl iodide", "Sodium hydroxide", "Chloroform", "Hydrogen gas", "Palladium on carbon", "Acetic acid", "Sodium borohydride", "Toluene" ], "Reaction": [ "Step 1: React quinoline with ethyl iodide in the presence of sodium hydroxide to form ethylquinoline.", "Step 2: React ethylquinoline with chloroform and hydrogen gas in the presence of palladium on carbon to form 9-ethyl-9-dehydroquinoline.", "Step 3: React 9-ethyl-9-dehydroquinoline with acetic acid to form 9-ethyl-9-dehydrocinchonine.", "Step 4: Reduce 9-ethyl-9-dehydrocinchonine using sodium borohydride in the presence of acetic acid to form cinchonidine.", "Step 5: Purify cinchonidine using toluene to obtain the final product." ] } | |
CAS No. |
485-71-2 |
Molecular Formula |
C19H22N2O |
Molecular Weight |
294.4 g/mol |
IUPAC Name |
(R)-[(4S,5R)-5-ethenyl-1-azabicyclo[2.2.2]octan-2-yl]-quinolin-4-ylmethanol |
InChI |
InChI=1S/C19H22N2O/c1-2-13-12-21-10-8-14(13)11-18(21)19(22)16-7-9-20-17-6-4-3-5-15(16)17/h2-7,9,13-14,18-19,22H,1,8,10-12H2/t13-,14-,18?,19+/m0/s1 |
InChI Key |
KMPWYEUPVWOPIM-CZHSWUJBSA-N |
Isomeric SMILES |
C=C[C@H]1CN2CC[C@H]1CC2[C@@H](C3=CC=NC4=CC=CC=C34)O |
SMILES |
C=CC1CN2CCC1CC2C(C3=CC=NC4=CC=CC=C34)O |
Canonical SMILES |
C=CC1CN2CCC1CC2C(C3=CC=NC4=CC=CC=C34)O |
melting_point |
210.5 °C |
118-10-5 485-71-2 1042167-24-7 |
|
physical_description |
Solid |
Pictograms |
Irritant; Health Hazard |
solubility |
0.2 mg/mL at 25 °C |
Synonyms |
2-Quinuclidinemethanol, alpha-4-quinolyl-5-vinyl- cinchonidine cinchonidine hydrochloride cinchonidine monohydrochloride cinchonidine sulfate(2:1) cinchonidine, (1beta,3alpha,4beta,8alpha,9R)-isome |
Origin of Product |
United States |
Retrosynthesis Analysis
AI-Powered Synthesis Planning: Our tool employs the Template_relevance Pistachio, Template_relevance Bkms_metabolic, Template_relevance Pistachio_ringbreaker, Template_relevance Reaxys, Template_relevance Reaxys_biocatalysis model, leveraging a vast database of chemical reactions to predict feasible synthetic routes.
One-Step Synthesis Focus: Specifically designed for one-step synthesis, it provides concise and direct routes for your target compounds, streamlining the synthesis process.
Accurate Predictions: Utilizing the extensive PISTACHIO, BKMS_METABOLIC, PISTACHIO_RINGBREAKER, REAXYS, REAXYS_BIOCATALYSIS database, our tool offers high-accuracy predictions, reflecting the latest in chemical research and data.
Strategy Settings
Precursor scoring | Relevance Heuristic |
---|---|
Min. plausibility | 0.01 |
Model | Template_relevance |
Template Set | Pistachio/Bkms_metabolic/Pistachio_ringbreaker/Reaxys/Reaxys_biocatalysis |
Top-N result to add to graph | 6 |
Feasible Synthetic Routes
Q1: What is the role of cinchonidine in heterogeneous catalysis?
A: this compound is a chiral modifier frequently employed in heterogeneous catalysis, especially in the enantioselective hydrogenation of prochiral ketones and α,β-unsaturated carboxylic acids. [, , , , , ] When adsorbed on metal surfaces like platinum or palladium, it creates a chiral environment that favors the formation of one enantiomer over the other. [, , ]
Q2: How does this compound adsorb on metal surfaces like platinum?
A: this compound adsorbs on platinum surfaces primarily via its quinoline moiety. [, , ] The adsorption mode is coverage-dependent, with multiple species coexisting on the surface. [, , ] At low concentrations, a predominantly flat adsorption mode is observed, whereas at higher concentrations, tilted species, including α-H abstracted and N lone pair bonded this compound, emerge. [, , ]
Q3: What is the significance of the different adsorption modes of this compound?
A: The different adsorption modes of this compound on platinum surfaces have significant implications for enantioselectivity. [] It is suggested that the flat-lying orientation of the quinoline ring, prevalent at intermediate concentrations, is associated with optimal activity and enantioselectivity in catalytic processes. []
Q4: How does the choice of solvent impact this compound's behavior in heterogeneous catalysis?
A: The solvent plays a crucial role in this compound adsorption and catalytic performance. [, ] Non-polar solvents, such as cyclohexane, allow adsorbed this compound to remain on the platinum surface, while more polar solvents, like dichloromethane, facilitate its removal. [] This behavior correlates with this compound solubility and the overall catalytic performance. [] For example, in the hydrogenation of ethyl pyruvate, ethanol as a solvent leads to higher enantioselectivity in a fixed-bed reactor compared to acetic acid, while the opposite is observed in a batch reactor. []
Q5: What is the role of hydrogen in this compound adsorption and catalytic activity?
A: Hydrogen plays a critical role in preparing effective this compound-modified platinum catalysts. [, ] Initially, it conditions the surface by reducing and removing contaminants, facilitating this compound adsorption. [, ] Subsequently, hydrogen can hydrogenate the quinoline fragment of this compound, promoting its desorption. [] Catalysts prepared and used under strictly anaerobic conditions display negligible activity and enantioselectivity, highlighting the importance of oxygen in successful catalyst preparation. []
Q6: How does the size of platinum particles affect this compound's adsorption and catalytic activity?
A: The size of platinum particles influences the distribution of different this compound conformations on the surface. [, ] Smaller particles, or those where platinum domains are reduced by alloying with gold, tend to disfavor the formation of flat-lying this compound species. [, ] This can impact the enantioselectivity of the catalyst.
Q7: Can this compound be immobilized on a support for heterogeneous catalysis?
A: Yes, this compound can be immobilized on supports like silica for heterogeneous catalysis. [, ] One strategy involves functionalizing this compound with a triethoxysilane group for subsequent attachment to silica. [] This immobilization can lead to catalysts with comparable or even superior performance to systems where this compound is added directly to the reaction mixture. []
Q8: Are there other molecules besides this compound used as chiral modifiers in heterogeneous catalysis?
A: Yes, various molecules besides this compound are used as chiral modifiers, including O-phenyl derivatives of this compound, (R)-2-(pyrrolidin-1-yl)-1-(1-naphthyl)ethanol (PNE), and synthetic ligands like quiphos. [, , ] The structure and properties of the modifier can significantly impact the adsorption mode and the resulting enantioselectivity of the catalyst. [, ]
Q9: What is the molecular formula and weight of this compound?
A9: The molecular formula of this compound is C19H22N2O, and its molecular weight is 294.38 g/mol.
Q10: What spectroscopic techniques are typically used to characterize this compound and its interactions?
A10: Several spectroscopic techniques are employed to characterize this compound, including:
- Nuclear Magnetic Resonance (NMR): This technique is essential for determining the structure, conformation, and interactions of this compound in solution. [, , , , ] It provides information about the spatial arrangement of atoms, hydrogen bonding, and the presence of different conformers. [, , , , ]
- Infrared Spectroscopy (IR): IR spectroscopy, including attenuated total reflection infrared (ATR-IR) spectroscopy, is used to study the adsorption of this compound on metal surfaces. [, , , , , ] It provides insights into the binding modes of this compound and its interactions with the surface and other molecules. [, , , , , ]
- Surface-Enhanced Raman Spectroscopy (SERS): This technique provides enhanced sensitivity for studying adsorbed species on metal surfaces, enabling the investigation of this compound's orientation and interactions on platinum. [, ]
Q11: How does modification of the this compound structure affect its activity and selectivity?
A: Modifying the this compound structure can significantly impact its performance as a chiral modifier. [, , , , , ] For example:
- C9-O-substitution: Introducing bulky substituents at the C9-OH group can lead to lower enantioselectivity and even reverse the enantiomeric preference in some cases. []
- Quinuclidine nitrogen modification: Altering the quinuclidine nitrogen, such as through quaternization, can impact its interaction with the substrate and the metal surface, influencing both activity and selectivity. [, , ]
- Hydrogenation of the quinoline ring: Hydrogenating the quinoline ring can affect its adsorption geometry and interaction with the metal surface, impacting the enantioselectivity. [, ]
Q12: What is the role of computational chemistry in understanding this compound's behavior?
A: Computational chemistry, particularly Density Functional Theory (DFT) calculations, has proven valuable in understanding this compound's behavior. [, , , , ] These calculations provide insights into:
- Conformational analysis: Identifying and analyzing the stability of different this compound conformations in solution and on surfaces. [, , , ]
- Adsorption energies: Determining the strength of interaction between this compound and metal surfaces in various orientations. [, ]
- Mechanistic understanding: Proposing and evaluating models for the interaction of this compound with substrates like pyruvate and the origin of enantioselectivity in catalytic reactions. []
Q13: What are some other applications of this compound beyond heterogeneous catalysis?
A13: Apart from its role in catalysis, this compound finds applications in:
- Chiral resolution: It can be used as a resolving agent to separate enantiomers of chiral compounds through the formation of diastereomeric salts. []
Q14: What analytical techniques are used to quantify this compound?
A: High-performance liquid chromatography (HPLC) is commonly employed for the quantification of this compound in various matrices. [, , ] This technique allows for separation and detection of this compound, even in complex mixtures.
Q15: What are some of the challenges and future directions in this compound research?
A15: Despite extensive research, several challenges and exciting research directions remain in the field of this compound, including:
- Elucidating the precise mechanism of enantioselection: While various models have been proposed, the exact mechanism by which this compound induces enantioselectivity in heterogeneous catalysis is still debated and requires further investigation. [, ]
- Designing more efficient and selective catalysts: Developing new synthetic methodologies and strategies to immobilize this compound on various supports with improved control over its orientation and accessibility could lead to more efficient and selective catalysts. []
- Exploring alternative applications: Given its diverse properties, investigating other potential applications of this compound, particularly in the biomedical field, holds promise. [, ] This requires a deeper understanding of its biological activity, toxicity, and pharmacokinetic properties.
Disclaimer and Information on In-Vitro Research Products
Please be aware that all articles and product information presented on BenchChem are intended solely for informational purposes. The products available for purchase on BenchChem are specifically designed for in-vitro studies, which are conducted outside of living organisms. In-vitro studies, derived from the Latin term "in glass," involve experiments performed in controlled laboratory settings using cells or tissues. It is important to note that these products are not categorized as medicines or drugs, and they have not received approval from the FDA for the prevention, treatment, or cure of any medical condition, ailment, or disease. We must emphasize that any form of bodily introduction of these products into humans or animals is strictly prohibited by law. It is essential to adhere to these guidelines to ensure compliance with legal and ethical standards in research and experimentation.