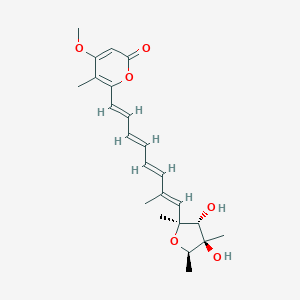
Citreoviridin
Overview
Description
Citreoviridin is a mycotoxin produced by various species of fungi, including Penicillium citreonigrum, Aspergillus terreus, and Eupenicillium ochrosalmoneum . It is commonly found as a contaminant in rice, corn, cereals, and meat products stored in damp conditions . This compound is known for its toxic effects, including causing cardiac beri beri, a disease characterized by heart failure .
Mechanism of Action
- Specifically, Citreoviridin interacts with the β subunit of F1Fo ATP synthase . This interaction disrupts ATP synthesis, affecting energy production within the cell.
- The inhibition of ATP production impacts various cellular processes, including metabolism, cell signaling, and growth .
- This compound alters basic metabolic processes, such as carbohydrate metabolism, mitochondrial functions, lipid and steroid metabolism, and protein biosynthesis .
- Impact on Bioavailability :
Target of Action
Mode of Action
Biochemical Pathways
Pharmacokinetics
Action Environment
Biochemical Analysis
Biochemical Properties
Citreoviridin acts as a potent inhibitor of the soluble mitochondrial ATPase (adenosine triphosphatase), similar to the closely related aurovertins B and D . It inhibits several mitochondrial energy-linked reactions, including ADP-stimulated respiration in whole mitochondria from ox heart and rat liver, ATP-driven reduction of NAD+ by succinate, ATP-driven NAD transhydrogenase, and ATPase from ox heart submitochondrial particles .
Cellular Effects
This compound has been shown to induce autophagy-dependent apoptosis through the lysosomal-mitochondrial axis in human liver HepG2 cells . It also significantly augments atherosclerotic plaques and increases expressions of ICAM-1, VCAM-1, VEGF, and ET-1 in aorta . The activation of NF-κB was significantly elevated by this compound treatment, indicating its effect on atherosclerosis may be regulated by activation of NF-κB .
Molecular Mechanism
This compound’s molecular mechanism of action involves the inhibition of the mitochondrial ATPase, leading to disruption of energy-linked reactions . It also induces apoptosis through the lysosomal-mitochondrial axis in HepG2 cells . Furthermore, it upregulates inflammation and endothelial dysfunction via the activation of NF-κB .
Temporal Effects in Laboratory Settings
It has been suggested that this compound is easily absorbed in swine and persists in the body for a long duration due to its slow metabolism .
Dosage Effects in Animal Models
It has been reported that this compound has a high bioavailability in swine .
Metabolic Pathways
The metabolic pathways of this compound involve hydroxylation, methylation, desaturation, and dihydroxylation . The expression of these four homologous genes were highly correlated with this compound production, suggesting that they may play an important role in this compound biosynthesis .
Transport and Distribution
This compound has a large volume of distribution and a long elimination half-life in intravenous administration, suggesting the possibility of a slow metabolism of this compound .
Subcellular Localization
Given its role as an inhibitor of mitochondrial ATPase, it is likely that this compound localizes to the mitochondria where it exerts its effects .
Preparation Methods
Synthetic Routes and Reaction Conditions: Citreoviridin is a highly reduced polyketide product. Its synthesis involves the use of polyketide synthases, which are enzymes that catalyze the formation of polyketides through the polymerization of acetyl and malonyl subunits . The biosynthetic pathway includes several steps, such as hydroxylation, methylation, desaturation, and dihydroxylation .
Industrial Production Methods: Industrial production of this compound typically involves the cultivation of Penicillium citreonigrum under controlled conditions to maximize the yield of the mycotoxin. The fungi are grown in a nutrient-rich medium, and the this compound is extracted and purified using various chromatographic techniques .
Chemical Reactions Analysis
Types of Reactions: Citreoviridin undergoes several types of chemical reactions, including oxidation, reduction, and substitution. These reactions are facilitated by various reagents and conditions.
Common Reagents and Conditions:
Oxidation: this compound can be oxidized using reagents such as hydrogen peroxide or potassium permanganate.
Reduction: Reduction reactions can be carried out using reducing agents like sodium borohydride or lithium aluminum hydride.
Substitution: Substitution reactions often involve nucleophiles such as amines or thiols.
Major Products: The major products formed from these reactions include hydroxylated, methylated, and desaturated derivatives of this compound .
Scientific Research Applications
Citreoviridin has several scientific research applications across various fields:
Chemistry:
- Used as a model compound to study the biosynthesis of polyketides.
- Employed in the development of synthetic methods for polyketide synthesis .
Biology:
- Investigated for its toxic effects on cellular processes, including its ability to inhibit mitochondrial ATP synthetase .
- Studied for its role in inducing autophagy-dependent apoptosis in human liver cells .
Medicine:
- Explored for its potential use in developing treatments for diseases caused by fungal contamination.
- Used in research to understand the mechanisms of mycotoxin-induced diseases .
Industry:
- Applied in the development of detection methods for mycotoxins in food products.
- Utilized in the study of fungal contamination in agricultural products .
Comparison with Similar Compounds
Citreoviridin belongs to a class of F1-ATPase β-subunit inhibitors that share an α-pyrone polyene structure. Similar compounds include:
Aurovertin: Another polyketide that inhibits mitochondrial ATP synthetase and has similar toxic effects.
Verrucosidin: A mycotoxin with a similar structure and mechanism of action.
Asteltoxin: Shares structural similarities and inhibits mitochondrial functions.
Uniqueness: this compound is unique due to its specific production by Penicillium citreonigrum and its potent inhibitory effects on mitochondrial ATP synthetase. Its ability to induce autophagy-dependent apoptosis also sets it apart from other similar compounds .
Properties
IUPAC Name |
6-[(1E,3E,5E,7E)-8-[(2S,3R,4R,5R)-3,4-dihydroxy-2,4,5-trimethyloxolan-2-yl]-7-methylocta-1,3,5,7-tetraenyl]-4-methoxy-5-methylpyran-2-one | |
---|---|---|
Source | PubChem | |
URL | https://pubchem.ncbi.nlm.nih.gov | |
Description | Data deposited in or computed by PubChem | |
InChI |
InChI=1S/C23H30O6/c1-15(14-22(4)21(25)23(5,26)17(3)29-22)11-9-7-8-10-12-18-16(2)19(27-6)13-20(24)28-18/h7-14,17,21,25-26H,1-6H3/b8-7+,11-9+,12-10+,15-14+/t17-,21+,22+,23+/m1/s1 | |
Source | PubChem | |
URL | https://pubchem.ncbi.nlm.nih.gov | |
Description | Data deposited in or computed by PubChem | |
InChI Key |
JLSVDPQAIKFBTO-OMCRQDLASA-N | |
Source | PubChem | |
URL | https://pubchem.ncbi.nlm.nih.gov | |
Description | Data deposited in or computed by PubChem | |
Canonical SMILES |
CC1C(C(C(O1)(C)C=C(C)C=CC=CC=CC2=C(C(=CC(=O)O2)OC)C)O)(C)O | |
Source | PubChem | |
URL | https://pubchem.ncbi.nlm.nih.gov | |
Description | Data deposited in or computed by PubChem | |
Isomeric SMILES |
C[C@@H]1[C@]([C@H]([C@](O1)(C)/C=C(\C)/C=C/C=C/C=C/C2=C(C(=CC(=O)O2)OC)C)O)(C)O | |
Source | PubChem | |
URL | https://pubchem.ncbi.nlm.nih.gov | |
Description | Data deposited in or computed by PubChem | |
Molecular Formula |
C23H30O6 | |
Source | PubChem | |
URL | https://pubchem.ncbi.nlm.nih.gov | |
Description | Data deposited in or computed by PubChem | |
DSSTOX Substance ID |
DTXSID301017584 | |
Record name | Citreoviridin | |
Source | EPA DSSTox | |
URL | https://comptox.epa.gov/dashboard/DTXSID301017584 | |
Description | DSSTox provides a high quality public chemistry resource for supporting improved predictive toxicology. | |
Molecular Weight |
402.5 g/mol | |
Source | PubChem | |
URL | https://pubchem.ncbi.nlm.nih.gov | |
Description | Data deposited in or computed by PubChem | |
CAS No. |
25425-12-1 | |
Record name | Citreoviridin | |
Source | CAS Common Chemistry | |
URL | https://commonchemistry.cas.org/detail?cas_rn=25425-12-1 | |
Description | CAS Common Chemistry is an open community resource for accessing chemical information. Nearly 500,000 chemical substances from CAS REGISTRY cover areas of community interest, including common and frequently regulated chemicals, and those relevant to high school and undergraduate chemistry classes. This chemical information, curated by our expert scientists, is provided in alignment with our mission as a division of the American Chemical Society. | |
Explanation | The data from CAS Common Chemistry is provided under a CC-BY-NC 4.0 license, unless otherwise stated. | |
Record name | Citreoviridin | |
Source | ChemIDplus | |
URL | https://pubchem.ncbi.nlm.nih.gov/substance/?source=chemidplus&sourceid=0025425121 | |
Description | ChemIDplus is a free, web search system that provides access to the structure and nomenclature authority files used for the identification of chemical substances cited in National Library of Medicine (NLM) databases, including the TOXNET system. | |
Record name | Citreoviridin | |
Source | EPA DSSTox | |
URL | https://comptox.epa.gov/dashboard/DTXSID301017584 | |
Description | DSSTox provides a high quality public chemistry resource for supporting improved predictive toxicology. | |
Record name | Citreoviridin | |
Source | European Chemicals Agency (ECHA) | |
URL | https://echa.europa.eu/information-on-chemicals | |
Description | The European Chemicals Agency (ECHA) is an agency of the European Union which is the driving force among regulatory authorities in implementing the EU's groundbreaking chemicals legislation for the benefit of human health and the environment as well as for innovation and competitiveness. | |
Explanation | Use of the information, documents and data from the ECHA website is subject to the terms and conditions of this Legal Notice, and subject to other binding limitations provided for under applicable law, the information, documents and data made available on the ECHA website may be reproduced, distributed and/or used, totally or in part, for non-commercial purposes provided that ECHA is acknowledged as the source: "Source: European Chemicals Agency, http://echa.europa.eu/". Such acknowledgement must be included in each copy of the material. ECHA permits and encourages organisations and individuals to create links to the ECHA website under the following cumulative conditions: Links can only be made to webpages that provide a link to the Legal Notice page. | |
Record name | CITREOVIRIDIN | |
Source | FDA Global Substance Registration System (GSRS) | |
URL | https://gsrs.ncats.nih.gov/ginas/app/beta/substances/OWX7Q6CF4F | |
Description | The FDA Global Substance Registration System (GSRS) enables the efficient and accurate exchange of information on what substances are in regulated products. Instead of relying on names, which vary across regulatory domains, countries, and regions, the GSRS knowledge base makes it possible for substances to be defined by standardized, scientific descriptions. | |
Explanation | Unless otherwise noted, the contents of the FDA website (www.fda.gov), both text and graphics, are not copyrighted. They are in the public domain and may be republished, reprinted and otherwise used freely by anyone without the need to obtain permission from FDA. Credit to the U.S. Food and Drug Administration as the source is appreciated but not required. | |
Retrosynthesis Analysis
AI-Powered Synthesis Planning: Our tool employs the Template_relevance Pistachio, Template_relevance Bkms_metabolic, Template_relevance Pistachio_ringbreaker, Template_relevance Reaxys, Template_relevance Reaxys_biocatalysis model, leveraging a vast database of chemical reactions to predict feasible synthetic routes.
One-Step Synthesis Focus: Specifically designed for one-step synthesis, it provides concise and direct routes for your target compounds, streamlining the synthesis process.
Accurate Predictions: Utilizing the extensive PISTACHIO, BKMS_METABOLIC, PISTACHIO_RINGBREAKER, REAXYS, REAXYS_BIOCATALYSIS database, our tool offers high-accuracy predictions, reflecting the latest in chemical research and data.
Strategy Settings
Precursor scoring | Relevance Heuristic |
---|---|
Min. plausibility | 0.01 |
Model | Template_relevance |
Template Set | Pistachio/Bkms_metabolic/Pistachio_ringbreaker/Reaxys/Reaxys_biocatalysis |
Top-N result to add to graph | 6 |
Feasible Synthetic Routes
A: Citreoviridin is a potent inhibitor of mitochondrial F1F0-ATP synthase, specifically targeting the β-subunit. [] This interaction disrupts ATP synthesis, a critical process for cellular energy production. [, ] Downstream effects include:
- Inhibition of mitochondrial energy-linked reactions: This includes ADP-stimulated respiration, ATP-driven reduction of NAD+, and ATP-driven NAD transhydrogenase. []
- Induction of the unfolded protein response (UPR): This cellular stress response is triggered by the accumulation of unfolded proteins, leading to cell cycle arrest and inhibition of proliferation. []
- Increased reactive oxygen species (ROS) production: This contributes to oxidative stress, further enhancing UPR and leading to cell death. []
- Lysosomal membrane permeabilization: This releases cathepsin D, a protease that contributes to apoptosis. []
- Mitochondrial transmembrane potential collapse: This is a hallmark of mitochondrial dysfunction and contributes to apoptosis. []
ANone: this compound's structural characteristics are:
- Molecular Formula: C23H30O6 [, ]
- Molecular Weight: 402.48 g/mol [, ]
- Spectroscopic data: this compound exhibits characteristic UV/Vis, FT-IR, 1H-NMR, 13C-NMR, LC-MS/MS, and LC-MSD TOF spectral data. [, , ]
A: this compound itself doesn't possess catalytic properties. Its primary mode of action is the inhibition of F1F0-ATP synthase. [, ]
A: While computational studies specifically on this compound are limited in the provided research, mathematical modeling has been used to construct response networks illustrating the relationship between phosphorylated heat shock protein 90 β and the mitogen-activated protein kinase signaling pathway following this compound treatment. []
A: Modifications to this compound's structure, such as acetylation, decrease its inhibitory potency against F1F0-ATPase. [] Monoacetylation has a less pronounced effect compared to diacetylation, highlighting the importance of specific functional groups for activity. [] Hydrogenation of this compound monoacetate also diminishes its inhibitory potency. []
A: this compound shows good stability when stored frozen and protected from light for up to 10 months. [] Isomerization can occur with prolonged storage. [] Specific formulation strategies to enhance stability, solubility, or bioavailability are not discussed in the provided research.
A: Studies in swine revealed high bioavailability of this compound (116.4%) and a long elimination half-life (17.7 ± 3.3 h), suggesting slow metabolism. [] In vitro studies using Caco-2 cells indicated high intestinal permeability, suggesting easy absorption in the human intestine. [, ]
ANone: The provided research doesn't discuss resistance mechanisms to this compound or its potential cross-resistance with other compounds.
A: this compound is a mycotoxin with established toxicity. * Animal studies: In mice, near-lethal doses cause decreased motor activity, hypothermia, and catalepsy. [] Intravenous administration in rabbits leads to respiratory and cardiovascular failure. []* Cellular effects: this compound induces mitochondrial damage, leading to swelling, cristae breakage, and vacuolation. [] It also causes DNA damage in a dose-dependent manner. [] * Human health concerns: this compound is linked to acute cardiac beriberi, a potentially fatal condition. [, ]
ANone: The provided research does not discuss specific drug delivery or targeting strategies for this compound.
A: While specific biomarkers for this compound's effects are not identified in the provided research, DNA damage assessed by the comet assay (single-cell gel electrophoresis) has been explored as a potential biomarker for epidemiological studies. []
A: Various analytical techniques are employed for this compound analysis:* Chromatographic techniques: High-performance liquid chromatography (HPLC) coupled with various detectors like UV/Vis (PDA), fluorescence, and mass spectrometry (MS/MS, TOF) are widely used. [, , , , , , ] * Spectroscopic techniques: UV/Vis, FT-IR, 1H-NMR, and 13C-NMR are utilized for structural characterization. [, , ]* Thin-layer chromatography (TLC): This technique is used for rapid detection and quantification. []
ANone: The provided research doesn't provide information about the environmental impact or degradation of this compound.
ANone: The provided research primarily focuses on this compound's extraction, purification, and analysis, without specific details on its dissolution or solubility in various media.
A: Analytical methods for this compound detection and quantification, particularly HPLC-based methods, are validated for parameters like linearity, sensitivity (limit of detection), recovery, and precision (repeatability). [, , , , ]
ANone: The provided research focuses on the analytical aspects and biological effects of this compound without delving into quality control and assurance measures during development, manufacturing, or distribution.
A: this compound research has a significant history:* 1947: First isolated in Japan during investigations of "yellow rice" disease, linked to cardiac beriberi. [, ]* 1970s - 1980s: Studies focused on elucidating its structure, biosynthetic pathway, and mechanism of action as an F1F0-ATPase inhibitor. [, , ]* 2006: Implicated in a beriberi outbreak in Brazil, renewing interest in its toxicology and occurrence in food. []* Recent years: Research focuses on understanding its molecular mechanisms of toxicity, potential as an anti-cancer agent, and developing sensitive analytical methods for detection. [, , , , , ]
ANone: this compound research exemplifies cross-disciplinary collaboration:
- Mycology and Food Science: Studying this compound-producing fungi, contamination routes in crops, and developing strategies for prevention. [, , , ]
- Analytical Chemistry: Developing and validating sensitive, specific, and robust methods for this compound detection and quantification in various matrices. [, , , , ]
- Toxicology and Pharmacology: Investigating this compound's mechanisms of toxicity, target organs, and potential long-term health effects. [, , , ]
- Biochemistry and Molecular Biology: Elucidating this compound's interaction with F1F0-ATPase, downstream signaling pathways, and cellular responses. [, , , , ]
- Medicinal Chemistry: Exploring this compound's potential as a lead compound for developing novel therapeutics, particularly in cancer and diseases involving ectopic ATP synthase. [, ]
Disclaimer and Information on In-Vitro Research Products
Please be aware that all articles and product information presented on BenchChem are intended solely for informational purposes. The products available for purchase on BenchChem are specifically designed for in-vitro studies, which are conducted outside of living organisms. In-vitro studies, derived from the Latin term "in glass," involve experiments performed in controlled laboratory settings using cells or tissues. It is important to note that these products are not categorized as medicines or drugs, and they have not received approval from the FDA for the prevention, treatment, or cure of any medical condition, ailment, or disease. We must emphasize that any form of bodily introduction of these products into humans or animals is strictly prohibited by law. It is essential to adhere to these guidelines to ensure compliance with legal and ethical standards in research and experimentation.