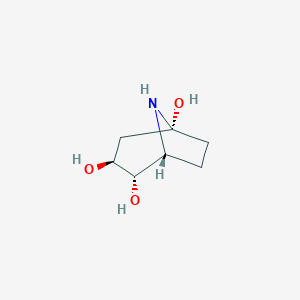
Calystegine A5
Overview
Description
Calystegine A5 is a nortropane alkaloid characterized by a bicyclic structure composed of carbon, hydrogen, oxygen, and nitrogen atoms. It belongs to the A-group calystegines, which share a common skeleton but differ in hydroxyl (-OH) and amino (-NH) substituent positions . This compound is naturally abundant in plants of the Solanaceae family, such as Hyoscyamus albus and Solanum tuberosum (potato).
Preparation Methods
Extraction Methodologies for Calystegine A5
The isolation of this compound from plant matrices requires optimized extraction protocols to balance recovery efficiency and selectivity. Recent studies have compared methanol-based solvents with aqueous mixtures. For instance, a 50:50 (v/v) methanol-water solution demonstrated superior recovery rates (73.7–106%) for calystegines in tomato varieties compared to methanol with 1% formic acid, which exhibited variability due to matrix effects . The extraction process typically involves homogenizing plant material (1–10 g) in solvent, followed by centrifugation and filtration. A reduction in sample size to 1 g was found to maintain recovery efficiency while simplifying subsequent steps . Post-extraction, evaporation under nitrogen or lyophilization concentrates the alkaloid fraction, preparing it for purification .
Purification Techniques
Purification of this compound relies on sequential ion-exchange chromatography to remove interfering compounds. Cation-exchange resins such as Amberlite IR 120B (H⁺ form) effectively capture protonated alkaloids, while anion-exchange columns (e.g., Dowex 1×2, Cl⁻ form) eliminate anionic contaminants . This dual-column approach ensures high purity, critical for downstream analytical applications. Capillary electrophoresis (CE) with borate buffers (80 mM sodium tetraborate, pH 9.2) has also been employed to separate calystegines, leveraging borate complexation to enhance resolution . The migration behavior of this compound in CE is influenced by its hydroxyl group configuration, enabling baseline separation from structurally similar analogs in under 12 minutes .
Derivatization Strategies for GC-Based Analysis
Due to its lack of UV chromophores, this compound requires derivatization for detection via GC-MS or GC-Q-Orbitrap. Trimethylsilylation using hexamethyldisilazane (HMDS) and trimethylchlorosilane (TMCS) in pyridine (250:40:10 μL) at 70°C for 30 minutes generates volatile trimethylsilyl (TMS) ether derivatives . This reaction substitutes hydroxyl groups with TMS moieties, improving thermal stability and ionization efficiency. Optimized derivatization conditions yield reproducible peaks with minimal byproducts, as evidenced by total ion chromatograms showing distinct signals for this compound at retention times between 8–12 minutes .
Table 1: Optimized Derivatization Conditions for this compound
Parameter | Optimal Value | Impact on Derivatization Efficiency |
---|---|---|
Pyridine Volume | 250 μL | Ensures complete dissolution |
HMDS Volume | 40 μL | Maximizes silylation |
TMCS Volume | 10 μL | Enhances reaction rate |
Temperature | 70°C | Accelerates derivatization |
Time | 30 minutes | Balances completeness and side reactions |
Biosynthetic Pathways and Enzymatic Regulation
This compound biosynthesis in A. belladonna involves cytochrome P450 enzymes, notably AbP450-5021 and AbP450-116623, which hydroxylate and demethylate tropane precursors . Virus-induced gene silencing (VIGS) of these enzymes in A. belladonna roots reduced this compound levels by 40–60%, while pseudotropine (a precursor) accumulated, confirming their role in late-stage modifications . This pathway diverges from hyoscyamine biosynthesis, as calystegines derive from pseudotropine rather than tropine, explaining their distinct stereochemistry and hydroxylation patterns .
Quantitative Analysis in Plant Varieties
GC-Q-Orbitrap analysis of tomato cultivars revealed significant variability in this compound content, with concentrations ranging from 0.65 mg/kg in 'Bernal A' to 12.47 mg/kg in 'Zoraida' . These disparities reflect genetic differences influencing alkaloid biosynthesis. Method validation demonstrated a limit of detection (LOD) of 0.25 mg/kg and a limit of quantification (LOQ) of 0.5 mg/kg, ensuring robust quantitation even in low-abundance samples .
Table 2: this compound Concentrations in Tomato Varieties
Variety | This compound (mg/kg) | Relative Abundance |
---|---|---|
Zoraida | 12.47 | High |
Satyplum | 9.82 | Moderate |
Bernal A | 0.65 | Low |
Method Validation and Quality Control
Intraday precision studies reported relative standard deviations (RSDs) ≤20% for this compound at concentrations ≥0.5 mg/kg, meeting AOAC guidelines for bioanalytical methods . Recovery rates from spiked tomato samples averaged 85–95%, affirming method accuracy . Capillary electrophoresis methods exhibited comparable precision, with migration time RSDs <1.5% and peak area RSDs <5% .
Chemical Reactions Analysis
Types of Reactions: Calystegine A5 undergoes various chemical reactions, including:
Oxidation: It can be oxidized under specific conditions, although detailed pathways are not extensively documented.
Reduction: Reduction reactions can modify its structure, potentially affecting its biological activity.
Substitution: Substitution reactions can occur, particularly involving its hydroxyl groups.
Common Reagents and Conditions: Common reagents used in these reactions include oxidizing agents like potassium permanganate and reducing agents like sodium borohydride. The conditions vary depending on the desired reaction and product.
Major Products: The major products formed from these reactions depend on the specific reagents and conditions used. For example, oxidation may lead to the formation of ketones or aldehydes, while reduction may yield alcohols.
Scientific Research Applications
Metabolic Activity Enhancement
Research has demonstrated that Calystegine A5 can significantly improve the metabolic activity of human adipose-derived stromal cells (HuASCs) under hyperglycemic conditions. A study indicated that treatment with calystegines, including A5, enhanced cell survival and reduced oxidative stress in HuASCs exposed to high glucose levels. The underlying mechanisms involve the modulation of cellular signaling pathways such as PI3K/AKT/mTOR, which are crucial for cell survival and metabolism .
Table 1: Effects of this compound on HuASCs
Parameter | Control Group | This compound (125 µg/mL) | This compound (250 µg/mL) |
---|---|---|---|
Cell Viability (%) | 100% | 85% | 90% |
Oxidative Stress Reduction (%) | - | 40% | 55% |
Inflammatory Response (Cytokines) | Low | Moderate | Low |
Antioxidant Properties
This compound exhibits potent antioxidant properties, which contribute to its protective effects against oxidative damage in cells. In vitro studies have shown that it can scavenge free radicals and enhance the endogenous antioxidant defense systems in cells subjected to oxidative stress. This capacity is particularly relevant in the context of diseases characterized by oxidative damage, such as diabetes and neurodegenerative disorders .
Anti-Inflammatory Effects
The anti-inflammatory potential of this compound has been explored through various studies. It has been shown to mitigate inflammatory responses in HuASCs by regulating the expression of pro-inflammatory cytokines. This effect is particularly beneficial in conditions like obesity and metabolic syndrome, where chronic inflammation plays a significant role .
Case Study: In Vivo Anti-Inflammatory Activity
A study involving extracts from Hyoscyamus albus, which contains this compound, demonstrated significant anti-inflammatory effects in animal models. The extracts inhibited edema formation induced by carrageenan, showcasing the compound's potential for therapeutic use in inflammatory conditions .
Glycosidase Inhibition
This compound is known for its ability to inhibit glycosidases, enzymes that play a critical role in carbohydrate metabolism. This inhibition can lead to altered carbohydrate absorption and metabolism, making it a candidate for managing conditions like diabetes by potentially lowering postprandial glucose levels .
Table 2: Inhibition Potency of Calystegines on Glycosidases
Glycosidase Type | Inhibition (%) at 100 µg/mL |
---|---|
α-Galactosidase | 70% |
β-Glucosidase | 65% |
β-Xylosidase | 60% |
Mechanism of Action
Calystegine A5 exerts its effects primarily by inhibiting glycosidases, enzymes that break down carbohydrates . This inhibition blocks carbohydrate metabolism, leading to various biological effects, including the reduction of oxidative and endoplasmic reticulum stress, inflammation, and the promotion of the PI3K/AKT/mTOR pathway . These pathways are crucial for cellular survival and metabolic functions.
Comparison with Similar Compounds
Comparative Analysis with Similar Compounds
Structural Classification
Calystegines are categorized into three groups based on structural features:
- A-group: Includes calystegines A3, A5, A6, and A7, characterized by a seven-membered nortropane ring with hydroxyl and amino substitutions.
- B-group : Comprises calystegines B1–B5, sharing a similar skeleton to the A-group but with distinct substitution patterns.
- N-group : Features calystegine N1, distinguished by an additional nitrogen atom in its structure .
Table 1: Structural Features of Select Calystegines
Compound | Group | Key Structural Differences |
---|---|---|
Calystegine A5 | A | Hydroxyl at C3, amino at C6 |
Calystegine A3 | A | Hydroxyl at C2, amino at C6 |
Calystegine B2 | B | Hydroxyl at C1, C2; amino at C6 |
Calystegine B4 | B | Hydroxyl at C1, C3; amino at C6 |
Calystegine N1 | N | Additional nitrogen in the bicyclic framework |
Occurrence and Abundance
This compound is frequently detected in Solanaceae plants but shows variable abundance across species and tissues:
- In Hyoscyamus albus seeds : this compound constitutes 45.91 µg/g dry weight (DW), significantly less than calystegine B4 (212.54 µg/g DW) but higher than A3 (54.9 µg/g DW) .
- In potatoes : A5 is detected in trace amounts (<1 mg/kg fresh weight), whereas calystegine A3 dominates (average 108.3 mg/kg) .
- In tomato products : A5 is present in all samples but often below the limit of quantification (LOQ = 0.5 mg/kg), unlike calystegine A3 (up to 19.0 mg/kg) .
Table 2: Comparative Abundance in Plant Tissues (Selected Data)
Source | This compound | Calystegine A3 | Calystegine B2 | Calystegine B4 |
---|---|---|---|---|
Hyoscyamus albus | 45.91 µg/g DW | 54.9 µg/g DW | 87.14 µg/g DW | 212.54 µg/g DW |
Potato tubers | <1 mg/kg f.w. | 108.3 mg/kg f.w. | 52.1 mg/kg f.w. | 3.7 mg/kg f.w. |
Tomato jam | <0.5 mg/kg | 19.0 mg/kg | 0.4 mg/kg | Not detected |
Biological Activity
Calystegine A5 is a polyhydroxylated nortropane alkaloid primarily found in various plants, including potatoes and tomatoes. Its biological activity has garnered attention due to its potential therapeutic applications, particularly in metabolic disorders, cancer, and inflammation. This article reviews the current understanding of the biological activity of this compound, highlighting its cytotoxic effects, metabolic benefits in diabetic conditions, and potential as an insecticide.
This compound is characterized by its unique structure, which allows it to mimic carbohydrate structures. This property is significant as it enables this compound to interact with various biological macromolecules, particularly glycosidases.
Cytotoxic Activity
Research indicates that this compound exhibits cytotoxic properties , making it a candidate for cancer research. In vitro studies have demonstrated that it can induce cell death in various cancer cell lines. The mechanism of action involves the inhibition of glycosidases, leading to disrupted carbohydrate metabolism and subsequent cellular dysfunction .
Table 1: Cytotoxicity of this compound on Different Cell Lines
Cell Line | IC50 (µM) | Assay Type |
---|---|---|
HeLa | 12.5 | MTT Assay |
MCF-7 | 15.0 | Colony Formation |
A549 | 10.0 | LDH Release |
Metabolic Activity in Diabetic Conditions
Recent studies have shown that this compound may improve the metabolic activity of human adipose-derived stem cells (hASCs) under hyperglycemic conditions. In a controlled experiment, treatment with this compound significantly enhanced cell survival and reduced oxidative stress in hASCs exposed to high glucose levels .
- Oxidative Stress Reduction : this compound promotes endogenous antioxidant defenses.
- Inflammation Modulation : It regulates pro-inflammatory cytokines and enhances anti-inflammatory responses.
- Signaling Pathways : The compound restores the PI3K/AKT/mTOR signaling pathway, crucial for cellular metabolism and survival .
Table 2: Effects of this compound on Human ASCs
Parameter | Control Group | This compound Group |
---|---|---|
Cell Viability (%) | 60 | 85 |
Oxidative Stress Marker (ROS) | 150 µM | 75 µM |
Inflammatory Cytokines (pg/mL) | 200 | 50 |
Anti-inflammatory Properties
This compound also exhibits anti-inflammatory activities . Studies involving extracts from Hyoscyamus albus containing calystegines demonstrated significant inhibition of carrageenan-induced paw edema in mice, showcasing the compound's potential for reducing inflammation .
Case Study: In Vivo Anti-inflammatory Effects
In a study conducted on mice:
- Dosage : Mice were treated with varying doses of calystegines extracted from Hyoscyamus albus.
- Results : The highest dose (200 mg/kg) resulted in a 58% reduction in edema compared to controls .
Insecticidal Properties
Some preliminary studies suggest that calystegines, including this compound, may possess insecticidal properties. However, further research is necessary to establish their efficacy and potential applications in pest control.
Q & A
Basic Research Questions
Q. What analytical techniques are most effective for identifying and quantifying Calystegine A5 in plant extracts?
this compound is commonly identified using gas chromatography-tandem mass spectrometry (GC-MS/MS) and capillary zone electrophoresis (CZE). For quantification, GC-MS/MS provides high sensitivity and specificity, especially when coupled with derivatization protocols to enhance volatility . Cross-validation with nuclear magnetic resonance (NMR) or high-performance liquid chromatography (HPLC) is recommended to confirm structural assignments and purity .
Q. How can researchers ensure reproducibility in protocols for isolating this compound from natural sources?
Reproducibility requires detailed documentation of extraction solvents (e.g., methanol-water mixtures), temperature conditions, and purification steps (e.g., column chromatography). Adhere to guidelines for experimental methods, such as those outlined in Reviews in Analytical Chemistry, which emphasize specifying equipment models, reagent sources, and validation metrics (e.g., recovery rates, limit of detection) .
Q. What structural features distinguish this compound from other nortropane alkaloids?
this compound is characterized by a bicyclic nortropane skeleton with hydroxyl groups at positions 1, 2, 3, 6, and 7, and an exocyclic amine. Unlike Calystegine B-series compounds, it lacks a hydroxyl group at position 4, which influences its glycosidase inhibition profile . Structural confirmation via X-ray crystallography or comparative NMR with reference standards is critical .
Advanced Research Questions
Q. How can contradictions in quantification data (e.g., percentage discrepancies in compositional studies) be resolved?
Discrepancies, such as the over-100% totals in some studies (e.g., ), may arise from normalization errors or overlapping chromatographic peaks. Mitigate this by:
- Using internal standards (e.g., deuterated analogs) for GC-MS/MS .
- Validating with orthogonal methods like HPLC coupled with evaporative light scattering detection (HPLC-ELSD) .
- Replicating experiments across independent labs to rule out systematic bias .
Q. What experimental designs are optimal for investigating structure-activity relationships (SAR) of this compound analogs?
SAR studies require synthesis of analogs with systematic modifications (e.g., hydroxyl group removal or stereochemical inversion). Pair this with in vitro assays (e.g., glycosidase inhibition) and molecular docking simulations. For example, compare this compound (1,2,3,6,7-pentahydroxy) with A3 (1,2,6,7-tetrahydroxy) to assess the role of position 3 hydroxylation . Include dose-response curves and statistical validation (e.g., ANOVA) to ensure robustness .
Q. What strategies are recommended for synthesizing this compound and its derivatives with high enantiomeric purity?
Multi-step enantioselective synthesis routes, such as Sharpless asymmetric dihydroxylation or enzymatic catalysis, are preferred. Reference protocols from J. Am. Chem. Soc. (e.g., Thalladi et al., 2000) for chiral pool strategies using tropinone precursors . Characterization via chiral HPLC and circular dichroism (CD) spectroscopy is essential to confirm enantiopurity .
Q. How should researchers address ethical considerations in publishing conflicting data on this compound bioactivity?
Follow the Beilstein Journal of Organic Chemistry guidelines:
- Disclose all raw data and statistical analyses in supplementary materials .
- Cite prior studies transparently, acknowledging methodological differences (e.g., cell lines, assay conditions) that may explain discrepancies .
- Avoid selective reporting; include negative results to provide a complete evidence base .
Q. Methodological Best Practices
Q. What interdisciplinary approaches enhance the study of this compound’s pharmacological potential?
Combine phytochemical isolation, synthetic chemistry, and molecular biology. For example:
- Use in silico tools (e.g., molecular dynamics) to predict target interactions.
- Validate predictions with in vivo models (e.g., diabetic zebrafish) and transcriptomic profiling .
- Cross-reference findings with ethnobotanical data to identify traditional uses (e.g., hyperglycemia management in Ericaceae plants) .
Q. How can researchers formulate hypotheses about this compound’s mechanisms of action?
Start with literature gaps, such as unresolved questions about its inhibition of α-glucosidases versus β-galactosidases. Design hypothesis-driven experiments:
- Compare IC₅₀ values across enzyme isoforms .
- Use knockout cell lines to isolate specific pathways (e.g., SIRT1/NF-κB/JNK in HepG2 cells) .
- Apply the "Relevant, Manageable, Appropriate" (RMA) framework for hypothesis refinement .
Q. What are the best practices for documenting synthetic procedures of this compound derivatives?
Follow Reviews in Analytical Chemistry standards:
- Report yields, reaction conditions (time, temperature), and characterization data (e.g., HRMS, ¹H/¹³C NMR) .
- Include failure analyses (e.g., side products from incomplete ring closure) to guide future optimizations .
- Deposit spectral data in public repositories (e.g., ChemSpider) for community verification .
Properties
IUPAC Name |
(1R,3S,4S,5R)-8-azabicyclo[3.2.1]octane-1,3,4-triol | |
---|---|---|
Source | PubChem | |
URL | https://pubchem.ncbi.nlm.nih.gov | |
Description | Data deposited in or computed by PubChem | |
InChI |
InChI=1S/C7H13NO3/c9-5-3-7(11)2-1-4(8-7)6(5)10/h4-6,8-11H,1-3H2/t4-,5+,6+,7-/m1/s1 | |
Source | PubChem | |
URL | https://pubchem.ncbi.nlm.nih.gov | |
Description | Data deposited in or computed by PubChem | |
InChI Key |
AXSWEYXUHSNDLV-JRTVQGFMSA-N | |
Source | PubChem | |
URL | https://pubchem.ncbi.nlm.nih.gov | |
Description | Data deposited in or computed by PubChem | |
Canonical SMILES |
C1CC2(CC(C(C1N2)O)O)O | |
Source | PubChem | |
URL | https://pubchem.ncbi.nlm.nih.gov | |
Description | Data deposited in or computed by PubChem | |
Isomeric SMILES |
C1C[C@]2(C[C@@H]([C@H]([C@@H]1N2)O)O)O | |
Source | PubChem | |
URL | https://pubchem.ncbi.nlm.nih.gov | |
Description | Data deposited in or computed by PubChem | |
Molecular Formula |
C7H13NO3 | |
Source | PubChem | |
URL | https://pubchem.ncbi.nlm.nih.gov | |
Description | Data deposited in or computed by PubChem | |
Molecular Weight |
159.18 g/mol | |
Source | PubChem | |
URL | https://pubchem.ncbi.nlm.nih.gov | |
Description | Data deposited in or computed by PubChem | |
CAS No. |
165905-26-0 | |
Record name | Calystegine A5 | |
Source | ChemIDplus | |
URL | https://pubchem.ncbi.nlm.nih.gov/substance/?source=chemidplus&sourceid=0165905260 | |
Description | ChemIDplus is a free, web search system that provides access to the structure and nomenclature authority files used for the identification of chemical substances cited in National Library of Medicine (NLM) databases, including the TOXNET system. | |
Retrosynthesis Analysis
AI-Powered Synthesis Planning: Our tool employs the Template_relevance Pistachio, Template_relevance Bkms_metabolic, Template_relevance Pistachio_ringbreaker, Template_relevance Reaxys, Template_relevance Reaxys_biocatalysis model, leveraging a vast database of chemical reactions to predict feasible synthetic routes.
One-Step Synthesis Focus: Specifically designed for one-step synthesis, it provides concise and direct routes for your target compounds, streamlining the synthesis process.
Accurate Predictions: Utilizing the extensive PISTACHIO, BKMS_METABOLIC, PISTACHIO_RINGBREAKER, REAXYS, REAXYS_BIOCATALYSIS database, our tool offers high-accuracy predictions, reflecting the latest in chemical research and data.
Strategy Settings
Precursor scoring | Relevance Heuristic |
---|---|
Min. plausibility | 0.01 |
Model | Template_relevance |
Template Set | Pistachio/Bkms_metabolic/Pistachio_ringbreaker/Reaxys/Reaxys_biocatalysis |
Top-N result to add to graph | 6 |
Feasible Synthetic Routes
Disclaimer and Information on In-Vitro Research Products
Please be aware that all articles and product information presented on BenchChem are intended solely for informational purposes. The products available for purchase on BenchChem are specifically designed for in-vitro studies, which are conducted outside of living organisms. In-vitro studies, derived from the Latin term "in glass," involve experiments performed in controlled laboratory settings using cells or tissues. It is important to note that these products are not categorized as medicines or drugs, and they have not received approval from the FDA for the prevention, treatment, or cure of any medical condition, ailment, or disease. We must emphasize that any form of bodily introduction of these products into humans or animals is strictly prohibited by law. It is essential to adhere to these guidelines to ensure compliance with legal and ethical standards in research and experimentation.