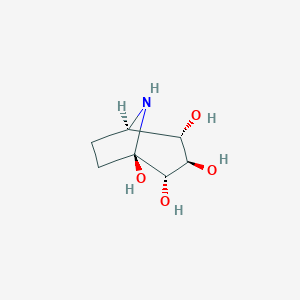
Calystegine B2
Overview
Description
Calystegine B2 is a naturally occurring tropane alkaloid found in various plants, particularly in the Solanaceae family. It is characterized by its nitrogenous bicyclic structure, specifically N-Methyl-8-azabicyclo[3.2.1]octane. This compound is known for its potent inhibitory effects on glycosidases, enzymes that hydrolyze glycosidic bonds in carbohydrates .
Preparation Methods
Synthetic Routes and Reaction Conditions: The synthesis of Calystegine B2 involves multiple steps, starting from carbohydrate derivatives. One notable method includes the use of D-xylose and L-arabinose derivatives. The key step in the synthesis is the construction of cycloheptanone via an intramolecular Nozaki–Hiyama–Kishi reaction of an aldehyde containing a Z-vinyl iodide . This reaction is followed by several steps, including Stork olefination and further transformations to yield this compound.
Industrial Production Methods: Industrial production of this compound is not widely documented, likely due to its complex synthesis and the availability of natural sources. advancements in synthetic organic chemistry may pave the way for more efficient industrial production methods in the future.
Chemical Reactions Analysis
Types of Reactions: Calystegine B2 undergoes various chemical reactions, including:
Oxidation: This reaction can modify the hydroxyl groups present in the molecule.
Reduction: Reduction reactions can alter the nitrogenous bicyclic structure.
Substitution: Substitution reactions can occur at the hydroxyl or amine groups.
Common Reagents and Conditions:
Oxidation: Common oxidizing agents include potassium permanganate and chromium trioxide.
Reduction: Reducing agents such as lithium aluminum hydride can be used.
Substitution: Reagents like alkyl halides or acyl chlorides can facilitate substitution reactions.
Major Products: The products formed from these reactions depend on the specific conditions and reagents used. For example, oxidation may yield ketones or aldehydes, while reduction can produce amines or alcohols.
Scientific Research Applications
Calystegine B2 has several scientific research applications:
Chemistry: It is used as a model compound to study glycosidase inhibition and to develop synthetic methodologies.
Biology: this compound is studied for its effects on glycosidase enzymes in various biological systems.
Industry: Its inhibitory properties are explored for applications in agriculture, particularly in pest control.
Mechanism of Action
Calystegine B2 exerts its effects primarily through the inhibition of glycosidase enzymes. It binds to the active site of these enzymes, preventing the hydrolysis of glycosidic bonds in carbohydrates. This inhibition can affect various biological processes, including carbohydrate metabolism and cell signaling pathways .
Comparison with Similar Compounds
- Calystegine B1
- Calystegine B3
- Calystegine A3
Comparison: Calystegine B2 is unique due to its specific inhibitory profile and its structural features. Compared to Calystegine B1 and B3, this compound has different stereochemistry, which influences its binding affinity and specificity towards glycosidases. Calystegine A3, on the other hand, has a different bicyclic structure, leading to variations in its biological activity .
Properties
IUPAC Name |
8-azabicyclo[3.2.1]octane-1,2,3,4-tetrol | |
---|---|---|
Details | Computed by Lexichem TK 2.7.0 (PubChem release 2021.05.07) | |
Source | PubChem | |
URL | https://pubchem.ncbi.nlm.nih.gov | |
Description | Data deposited in or computed by PubChem | |
InChI |
InChI=1S/C7H13NO4/c9-4-3-1-2-7(12,8-3)6(11)5(4)10/h3-6,8-12H,1-2H2 | |
Details | Computed by InChI 1.0.6 (PubChem release 2021.05.07) | |
Source | PubChem | |
URL | https://pubchem.ncbi.nlm.nih.gov | |
Description | Data deposited in or computed by PubChem | |
InChI Key |
FXFBVZOJVHCEDO-UHFFFAOYSA-N | |
Details | Computed by InChI 1.0.6 (PubChem release 2021.05.07) | |
Source | PubChem | |
URL | https://pubchem.ncbi.nlm.nih.gov | |
Description | Data deposited in or computed by PubChem | |
Canonical SMILES |
C1CC2(C(C(C(C1N2)O)O)O)O | |
Details | Computed by OEChem 2.3.0 (PubChem release 2021.05.07) | |
Source | PubChem | |
URL | https://pubchem.ncbi.nlm.nih.gov | |
Description | Data deposited in or computed by PubChem | |
Molecular Formula |
C7H13NO4 | |
Details | Computed by PubChem 2.1 (PubChem release 2021.05.07) | |
Source | PubChem | |
URL | https://pubchem.ncbi.nlm.nih.gov | |
Description | Data deposited in or computed by PubChem | |
Molecular Weight |
175.18 g/mol | |
Details | Computed by PubChem 2.1 (PubChem release 2021.05.07) | |
Source | PubChem | |
URL | https://pubchem.ncbi.nlm.nih.gov | |
Description | Data deposited in or computed by PubChem | |
CAS No. |
127414-85-1 | |
Record name | Calystegine B2 | |
Source | Human Metabolome Database (HMDB) | |
URL | http://www.hmdb.ca/metabolites/HMDB0038594 | |
Description | The Human Metabolome Database (HMDB) is a freely available electronic database containing detailed information about small molecule metabolites found in the human body. | |
Explanation | HMDB is offered to the public as a freely available resource. Use and re-distribution of the data, in whole or in part, for commercial purposes requires explicit permission of the authors and explicit acknowledgment of the source material (HMDB) and the original publication (see the HMDB citing page). We ask that users who download significant portions of the database cite the HMDB paper in any resulting publications. | |
Retrosynthesis Analysis
AI-Powered Synthesis Planning: Our tool employs the Template_relevance Pistachio, Template_relevance Bkms_metabolic, Template_relevance Pistachio_ringbreaker, Template_relevance Reaxys, Template_relevance Reaxys_biocatalysis model, leveraging a vast database of chemical reactions to predict feasible synthetic routes.
One-Step Synthesis Focus: Specifically designed for one-step synthesis, it provides concise and direct routes for your target compounds, streamlining the synthesis process.
Accurate Predictions: Utilizing the extensive PISTACHIO, BKMS_METABOLIC, PISTACHIO_RINGBREAKER, REAXYS, REAXYS_BIOCATALYSIS database, our tool offers high-accuracy predictions, reflecting the latest in chemical research and data.
Strategy Settings
Precursor scoring | Relevance Heuristic |
---|---|
Min. plausibility | 0.01 |
Model | Template_relevance |
Template Set | Pistachio/Bkms_metabolic/Pistachio_ringbreaker/Reaxys/Reaxys_biocatalysis |
Top-N result to add to graph | 6 |
Feasible Synthetic Routes
Q1: How does Calystegine B2 interact with its target enzymes?
A1: this compound acts as a potent competitive inhibitor of specific glycosidases, primarily α-galactosidase and β-glucosidase [, , , , ]. Its polyhydroxylated structure mimics the sugar moieties recognized by these enzymes, allowing it to bind to the active site and block substrate access [, ].
Q2: What is the molecular formula and weight of this compound?
A2: this compound has the molecular formula C8H15NO4 and a molecular weight of 189.21 g/mol.
Q3: Is there any spectroscopic data available for this compound?
A4: While the provided research excerpts do not delve into specific spectroscopic details, various techniques like nuclear magnetic resonance (NMR) and mass spectrometry (MS) have been employed to characterize this compound [, ]. These techniques provide valuable information regarding its structure, stereochemistry, and purity.
Q4: How does this compound perform under various conditions?
A4: The provided excerpts do not offer specific information on this compound's performance under different conditions (temperature, pH, etc.). Further investigation into its stability profile is necessary to determine its suitability for various applications.
Q5: Does this compound possess any catalytic properties?
A6: this compound is primarily recognized as a glycosidase inhibitor, not a catalyst. Its structure mimics the transition state of glycosidic bond hydrolysis, allowing it to bind tightly to the enzyme active site and prevent catalysis [, ].
Q6: Have computational methods been employed to study this compound?
A7: Yes, molecular docking studies have been conducted to understand the binding interactions of this compound with target enzymes like α-glucosidases []. These simulations provide insights into the molecular basis of its inhibitory activity. Further research using computational tools like quantitative structure-activity relationship (QSAR) modeling can help predict the activity of this compound analogs and guide the development of more potent and selective inhibitors [].
Q7: How do modifications to the this compound structure affect its activity and selectivity?
A8: Research indicates that even minor structural modifications significantly impact this compound's inhibitory profile. For instance, N-methylation enhances α-galactosidase inhibition but reduces activity towards other glycosidases, highlighting a shift in selectivity []. Introducing a hydroxyl group at the C6exo position, as seen in Calystegine B1 and C1, generally boosts inhibition of β-glucosidase and β-galactosidase while diminishing activity against α-galactosidase []. These findings underscore the importance of SAR studies in tailoring the properties of this compound analogs for specific therapeutic applications.
Disclaimer and Information on In-Vitro Research Products
Please be aware that all articles and product information presented on BenchChem are intended solely for informational purposes. The products available for purchase on BenchChem are specifically designed for in-vitro studies, which are conducted outside of living organisms. In-vitro studies, derived from the Latin term "in glass," involve experiments performed in controlled laboratory settings using cells or tissues. It is important to note that these products are not categorized as medicines or drugs, and they have not received approval from the FDA for the prevention, treatment, or cure of any medical condition, ailment, or disease. We must emphasize that any form of bodily introduction of these products into humans or animals is strictly prohibited by law. It is essential to adhere to these guidelines to ensure compliance with legal and ethical standards in research and experimentation.