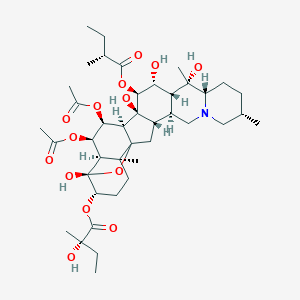
protoveratrine A
Overview
Description
Protoveratrine A is a naturally occurring steroidal alkaloid found in the Veratrum species, particularly Veratrum album. It is known for its potent biological activities, including its effects on the cardiovascular system. This compound has been used historically in medicine for its hypotensive properties, making it a compound of significant interest in both pharmacology and toxicology .
Mechanism of Action
Target of Action
Protoveratrine A, also known as Protoveratrin, is a potent alkaloid that primarily targets the cardiovascular system . It has been found to increase the release of gamma-amino[3H]butyrate from small slices of rat cerebral cortex . This effect increases with increasing protoveratrine concentration .
Mode of Action
This compound exerts its effects through its interaction with its targets. It has been found to be a potent activator of Na+ channels that are essential for neuronal signaling . If these ion channels are completely activated, the action potential from nerves to muscles are no longer transmitted leading to a complete arrest of cardiac and skeletal muscles .
Biochemical Pathways
It is known that the compound has a significant impact on the cardiovascular system, particularly in relation to hypertension and other cardiovascular diseases .
Pharmacokinetics
A liquid chromatographic coupled with tandem mass spectrometric method has been developed for the quantitation of trace amounts of this compound in rat plasma .
Result of Action
The molecular and cellular effects of this compound’s action primarily manifest as cardiotoxic effects . Patients exposed to this compound exhibit symptoms such as nausea, muscle weakness, bradycardia, and arterial hypotension . These symptoms are believed to be a result of this compound’s inhibition of the cardiac sodium channel NaV1.5 .
Biochemical Analysis
Biochemical Properties
Protoveratrine A plays a crucial role in various biochemical reactions. It interacts with several enzymes, proteins, and other biomolecules. One of the primary interactions is with the cardiac sodium channel NaV1.5, where this compound acts as an inhibitor . This interaction is significant as it influences the ion exchange across the cardiac cells, leading to its cardiotoxic effects. Additionally, this compound has been shown to affect the efflux of potassium ions from muscle and nerve cells, further highlighting its role in ion transport and cellular excitability .
Cellular Effects
This compound exerts profound effects on various cell types and cellular processes. In muscle and nerve cells, it increases the uptake of potassium ions and the release of calcium ions, which are critical for muscle contraction and nerve transmission . In cardiac cells, this compound’s inhibition of the NaV1.5 channel leads to altered cardiac rhythms and potential bradycardia . Furthermore, this compound has been observed to influence cell signaling pathways, gene expression, and cellular metabolism, contributing to its overall pharmacological profile.
Molecular Mechanism
At the molecular level, this compound exerts its effects primarily through its interaction with ion channels and enzymes. By binding to the NaV1.5 cardiac sodium channel, this compound inhibits the flow of sodium ions, which is essential for the generation and propagation of action potentials in cardiac cells . This inhibition leads to a decrease in cardiac excitability and can result in bradycardia and other cardiac dysfunctions. Additionally, this compound’s interaction with potassium and calcium ion channels further modulates cellular excitability and muscle contraction .
Temporal Effects in Laboratory Settings
In laboratory settings, the effects of this compound have been observed to change over time. Studies have shown that this compound remains stable in plasma for up to six months when stored at -80°C . Its effects on cellular function can vary over time. For instance, prolonged exposure to this compound can lead to sustained inhibition of ion channels, resulting in long-term alterations in cellular excitability and function . These temporal effects are crucial for understanding the compound’s pharmacokinetics and pharmacodynamics.
Dosage Effects in Animal Models
The effects of this compound vary significantly with different dosages in animal models. At lower doses, this compound has been shown to increase the sleep apnea index in Sprague-Dawley rats, indicating its impact on respiratory function . Higher doses, however, can lead to severe cardiotoxic effects, including bradycardia and hypotension . These dosage-dependent effects are critical for determining the therapeutic window and potential toxicity of this compound.
Metabolic Pathways
This compound is involved in several metabolic pathways. It is metabolized primarily in the liver, where it undergoes various biotransformation processes . The enzymes involved in its metabolism include cytochrome P450 enzymes, which facilitate the oxidation and subsequent conjugation of this compound. These metabolic processes are essential for the compound’s elimination from the body and its overall pharmacokinetic profile.
Transport and Distribution
Within cells and tissues, this compound is transported and distributed through various mechanisms. It interacts with transport proteins and binding proteins that facilitate its movement across cellular membranes . In the brain, this compound has been shown to inhibit the active transport of L-DOPA, indicating its potential impact on neurotransmitter regulation . Additionally, this compound’s distribution within tissues is influenced by its binding affinity to specific cellular components, affecting its localization and accumulation.
Subcellular Localization
This compound’s subcellular localization plays a significant role in its activity and function. It is primarily localized in the cytoplasm, where it interacts with various ion channels and enzymes . The compound’s localization is influenced by specific targeting signals and post-translational modifications that direct it to particular cellular compartments. Understanding this compound’s subcellular localization is crucial for elucidating its mechanism of action and potential therapeutic applications.
Preparation Methods
Synthetic Routes and Reaction Conditions
The synthesis of protoveratrine A involves complex organic reactions due to its intricate structure. Typically, the synthetic route includes multiple steps such as esterification, cyclization, and functional group modifications. The reaction conditions often require specific catalysts, controlled temperatures, and precise pH levels to ensure the correct formation of the desired product .
Industrial Production Methods
Industrial production of this compound is generally achieved through extraction from natural sources, particularly from the roots and rhizomes of Veratrum species. The extraction process involves solvent extraction followed by purification steps such as chromatography to isolate this compound in its pure form .
Chemical Reactions Analysis
Types of Reactions
Protoveratrine A undergoes various chemical reactions, including:
Oxidation: this compound can be oxidized to form different derivatives, which may have altered biological activities.
Reduction: Reduction reactions can modify the functional groups in this compound, potentially changing its pharmacological properties.
Substitution: This compound can undergo substitution reactions where specific atoms or groups in the molecule are replaced with others.
Common Reagents and Conditions
Common reagents used in the reactions of this compound include oxidizing agents like potassium permanganate, reducing agents such as lithium aluminum hydride, and various acids and bases for substitution reactions. The conditions for these reactions typically involve controlled temperatures and inert atmospheres to prevent unwanted side reactions .
Major Products Formed
The major products formed from the reactions of this compound depend on the type of reaction. For example, oxidation may yield hydroxylated derivatives, while reduction could produce deoxygenated compounds. Substitution reactions can result in a variety of analogs with different functional groups .
Scientific Research Applications
Protoveratrine A has a wide range of scientific research applications:
Chemistry: It is used as a reference compound in analytical chemistry for the development of detection and quantification methods.
Biology: this compound is studied for its effects on cellular processes and its potential as a tool in cell biology research.
Medicine: Historically used as an antihypertensive agent, this compound is now being investigated for its potential therapeutic applications in treating cardiovascular diseases and certain types of cancer.
Industry: This compound is used in the development of insecticides due to its toxic properties
Comparison with Similar Compounds
Protoveratrine A is part of a group of alkaloids found in Veratrum species, including protoveratrine B, jervine, and veratramine. Compared to these compounds, this compound is unique due to its specific structure and potent biological activity. Protoveratrine B, for example, is also a potent hypotensive agent but has a different molecular structure and slightly different pharmacological profile .
List of Similar Compounds
- Protoveratrine B
- Jervine
- Veratramine
- Veratridine
Properties
IUPAC Name |
[16,17-diacetyloxy-10,12,14,23-tetrahydroxy-6,10,19-trimethyl-13-(2-methylbutanoyloxy)-24-oxa-4-azaheptacyclo[12.12.0.02,11.04,9.015,25.018,23.019,25]hexacosan-22-yl] 2-hydroxy-2-methylbutanoate | |
---|---|---|
Details | Computed by Lexichem TK 2.7.0 (PubChem release 2021.05.07) | |
Source | PubChem | |
URL | https://pubchem.ncbi.nlm.nih.gov | |
Description | Data deposited in or computed by PubChem | |
InChI |
InChI=1S/C41H63NO14/c1-10-20(4)34(46)55-33-28(45)27-23(18-42-17-19(3)12-13-25(42)38(27,9)49)24-16-39-32(40(24,33)50)30(53-22(6)44)29(52-21(5)43)31-36(39,7)15-14-26(41(31,51)56-39)54-35(47)37(8,48)11-2/h19-20,23-33,45,48-51H,10-18H2,1-9H3 | |
Details | Computed by InChI 1.0.6 (PubChem release 2021.05.07) | |
Source | PubChem | |
URL | https://pubchem.ncbi.nlm.nih.gov | |
Description | Data deposited in or computed by PubChem | |
InChI Key |
HYTGGNIMZXFORS-UHFFFAOYSA-N | |
Details | Computed by InChI 1.0.6 (PubChem release 2021.05.07) | |
Source | PubChem | |
URL | https://pubchem.ncbi.nlm.nih.gov | |
Description | Data deposited in or computed by PubChem | |
Canonical SMILES |
CCC(C)C(=O)OC1C(C2C(CN3CC(CCC3C2(C)O)C)C4C1(C5C(C(C6C7(C5(C4)OC6(C(CC7)OC(=O)C(C)(CC)O)O)C)OC(=O)C)OC(=O)C)O)O | |
Details | Computed by OEChem 2.3.0 (PubChem release 2021.05.07) | |
Source | PubChem | |
URL | https://pubchem.ncbi.nlm.nih.gov | |
Description | Data deposited in or computed by PubChem | |
Molecular Formula |
C41H63NO14 | |
Details | Computed by PubChem 2.1 (PubChem release 2021.05.07) | |
Source | PubChem | |
URL | https://pubchem.ncbi.nlm.nih.gov | |
Description | Data deposited in or computed by PubChem | |
DSSTOX Substance ID |
DTXSID20861817 | |
Record name | 6,7-Bis(acetyloxy)-4,14,16,20-tetrahydroxy-15-[(2-methylbutanoyl)oxy]-4,9-epoxycevan-3-yl 2-hydroxy-2-methylbutanoate | |
Source | EPA DSSTox | |
URL | https://comptox.epa.gov/dashboard/DTXSID20861817 | |
Description | DSSTox provides a high quality public chemistry resource for supporting improved predictive toxicology. | |
Molecular Weight |
793.9 g/mol | |
Details | Computed by PubChem 2.1 (PubChem release 2021.05.07) | |
Source | PubChem | |
URL | https://pubchem.ncbi.nlm.nih.gov | |
Description | Data deposited in or computed by PubChem | |
Retrosynthesis Analysis
AI-Powered Synthesis Planning: Our tool employs the Template_relevance Pistachio, Template_relevance Bkms_metabolic, Template_relevance Pistachio_ringbreaker, Template_relevance Reaxys, Template_relevance Reaxys_biocatalysis model, leveraging a vast database of chemical reactions to predict feasible synthetic routes.
One-Step Synthesis Focus: Specifically designed for one-step synthesis, it provides concise and direct routes for your target compounds, streamlining the synthesis process.
Accurate Predictions: Utilizing the extensive PISTACHIO, BKMS_METABOLIC, PISTACHIO_RINGBREAKER, REAXYS, REAXYS_BIOCATALYSIS database, our tool offers high-accuracy predictions, reflecting the latest in chemical research and data.
Strategy Settings
Precursor scoring | Relevance Heuristic |
---|---|
Min. plausibility | 0.01 |
Model | Template_relevance |
Template Set | Pistachio/Bkms_metabolic/Pistachio_ringbreaker/Reaxys/Reaxys_biocatalysis |
Top-N result to add to graph | 6 |
Feasible Synthetic Routes
Q1: What is the primary mechanism of action of Protoveratrine A?
A1: this compound primarily acts by binding to voltage-gated sodium channels (NaV) in excitable cells like neurons and muscle cells [, , , ]. This binding keeps the channels open for a prolonged period, leading to persistent depolarization.
Q2: How does this compound's interaction with sodium channels affect nerve and muscle cells?
A2: The prolonged depolarization caused by this compound leads to repetitive firing in nerve cells and sustained contraction in muscle cells [, , , ]. This can result in various physiological effects, including hypotension and bradycardia.
Q3: this compound is known to induce bradycardia. How does this occur?
A3: this compound stimulates receptors in the nodose ganglion or nearby structures, triggering a reflex activation of sinus bradycardia []. This effect is primarily mediated through the vagus nerve, as vagotomy significantly reduces or abolishes bradycardia induced by this compound.
Q4: Does this compound directly affect blood vessels to lower blood pressure?
A4: While this compound can induce hypotension, studies suggest this effect is mainly due to reflex mechanisms rather than direct vasodilation [, , , ]. It is thought to activate baroreceptors, leading to a decrease in sympathetic outflow and subsequently lowering blood pressure.
Q5: What is the role of the hypothalamus in this compound's hypotensive effect?
A5: Research indicates that an intact and excitable hypothalamus is crucial for this compound to exert its hypotensive effect []. Inhibiting the hypothalamus with barbiturates or creating lesions in this brain region significantly diminishes this compound-induced hypotension.
Q6: this compound can induce the release of various neurotransmitters. Can you elaborate on this?
A6: this compound, especially in calcium-deficient conditions, enhances the release of gamma-aminobutyrate (GABA) from brain slices [, ]. It also affects the release of acetylcholine from nerve terminals in the Auerbach plexus of the guinea pig ileum, particularly at low stimulation frequencies [].
Q7: What is the molecular formula and weight of this compound?
A7: this compound has a molecular formula of C39H61NO13 and a molecular weight of 763.9 g/mol.
Q8: How do structural modifications to this compound affect its hypotensive potency?
A9: Research suggests that modifications to the ester group at the C3 position of this compound can significantly impact its hypotensive activity []. This highlights the importance of this structural feature for its pharmacological effects.
Q9: Do structural changes to the ester group at the C15 position also influence hypotensive activity?
A10: Yes, studies involving synthetic Protoverine tetraesters reveal that alterations to the C15 ester group can significantly affect hypotensive potency []. This emphasizes the importance of both the C3 and C15 ester groups for this compound's pharmacological profile.
Q10: What are the known toxic effects of this compound?
A11: this compound can cause various adverse effects, primarily related to its potent action on the cardiovascular and nervous systems [, , ]. These include nausea, vomiting, bradycardia, hypotension, and cardiac arrhythmias.
Q11: Is there a difference in toxicity between this compound and other Veratrum alkaloids?
A12: Yes, this compound demonstrates different toxicity profiles compared to other Veratrum alkaloids like Veratridine [, , , ]. For example, this compound is more potent in inducing bradycardia and hypotension than Veratridine.
Q12: What analytical techniques are commonly used to identify and quantify this compound?
A12: Common methods for analyzing this compound include:
- High-performance liquid chromatography coupled with tandem mass spectrometry (HPLC-MS/MS): This technique provides high sensitivity and selectivity for the identification and quantification of this compound in biological samples [, ].
- High-performance liquid chromatography with a diode-array detector (HPLC-DAD): This method, while less sensitive than HPLC-MS/MS, offers a more accessible option for quantifying this compound, especially when analyzing formulations like Veratrum aqua [].
Disclaimer and Information on In-Vitro Research Products
Please be aware that all articles and product information presented on BenchChem are intended solely for informational purposes. The products available for purchase on BenchChem are specifically designed for in-vitro studies, which are conducted outside of living organisms. In-vitro studies, derived from the Latin term "in glass," involve experiments performed in controlled laboratory settings using cells or tissues. It is important to note that these products are not categorized as medicines or drugs, and they have not received approval from the FDA for the prevention, treatment, or cure of any medical condition, ailment, or disease. We must emphasize that any form of bodily introduction of these products into humans or animals is strictly prohibited by law. It is essential to adhere to these guidelines to ensure compliance with legal and ethical standards in research and experimentation.