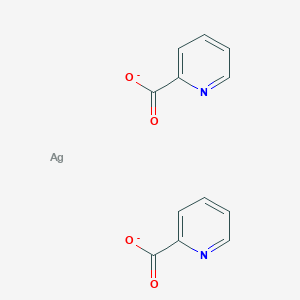
Silver(II) Pyridine-2-carboxylate
Overview
Description
Mechanism of Action
Target of Action
Silver(II) Pyridine-2-carboxylate primarily targets biological macromolecules and antioxidants . It has the potential to overcome microbial resistance by extensively attacking cellular components .
Mode of Action
This compound interacts with its targets by oxidizing them . The compound is more oxidizing than other silver compounds, which allows it to effectively interact with a variety of biological molecules .
Biochemical Pathways
The compound affects various biochemical pathways by oxidizing a wide variety of polar and nonpolar biological molecules . This includes antioxidants like glutathione, ascorbic acid, and vitamin E, unsaturated lipids like arachidonic and linoleic acids, carbohydrates like β-cyclodextrin, and proteins like cytochrome c .
Pharmacokinetics
The compound is known to be more stable in phosphate buffer than in dmso , which could potentially impact its absorption, distribution, metabolism, and excretion (ADME) properties.
Result of Action
The interaction of this compound with its targets results in various molecular and cellular effects. For instance, it oxidizes glutathione to its disulfide form . It also adds multiple oxygen atoms to unsaturated fatty acids, suggesting a radical mechanism . The compound completely oxidizes the seven hydroxyl groups of β-cyclodextrin to the corresponding carboxylates . Furthermore, its interaction with cytochrome c leads to protein aggregation and fragmentation, and dose-dependent oxidative damage .
Action Environment
The action of this compound can be influenced by environmental factors. For example, the compound is more stable in phosphate buffer than in DMSO , which suggests that its action, efficacy, and stability could be affected by the surrounding environment.
Preparation Methods
Synthetic Routes and Reaction Conditions: Silver(II) Pyridine-2-carboxylate can be synthesized through the reaction of silver nitrate with pyridine-2-carboxylic acid in the presence of a suitable base. The reaction typically occurs in an aqueous or alcoholic medium under controlled temperature and pH conditions .
Industrial Production Methods: While specific industrial production methods for this compound are not widely documented, the general approach involves optimizing the reaction conditions to achieve high purity and yield. This includes controlling the concentration of reactants, temperature, and pH, as well as employing purification techniques such as recrystallization .
Chemical Reactions Analysis
Types of Reactions: Silver(II) Pyridine-2-carboxylate undergoes various chemical reactions, including oxidation, reduction, and substitution reactions. It is commonly used as an oxidizing agent in synthetic reactions involving C-H bonds .
Common Reagents and Conditions:
Oxidation: this compound acts as an oxidant in reactions with primary amines or aldehydes in the presence of styrene.
Reduction: The compound can be reduced by antioxidants such as glutathione, ascorbic acid, and vitamin E.
Substitution: It can participate in substitution reactions with various organic ligands to form coordination complexes.
Major Products Formed: The major products formed from these reactions depend on the specific reactants and conditions used. For example, oxidation reactions with primary amines can yield amides, while reduction reactions with antioxidants result in the corresponding reduced forms .
Scientific Research Applications
Silver(II) Pyridine-2-carboxylate has a wide range of scientific research applications:
Comparison with Similar Compounds
- Silver(I) Pyridine-2-carboxylate
- Silver(II) 2,6-dicarboxypyridine complex
- Silver(I) complexes with carboxylate ligands
Properties
IUPAC Name |
pyridine-2-carboxylate;silver | |
---|---|---|
Source | PubChem | |
URL | https://pubchem.ncbi.nlm.nih.gov | |
Description | Data deposited in or computed by PubChem | |
InChI |
InChI=1S/2C6H5NO2.Ag/c2*8-6(9)5-3-1-2-4-7-5;/h2*1-4H,(H,8,9);/p-2 | |
Source | PubChem | |
URL | https://pubchem.ncbi.nlm.nih.gov | |
Description | Data deposited in or computed by PubChem | |
InChI Key |
ARXVTYGOLNYKET-UHFFFAOYSA-L | |
Source | PubChem | |
URL | https://pubchem.ncbi.nlm.nih.gov | |
Description | Data deposited in or computed by PubChem | |
Canonical SMILES |
C1=CC=NC(=C1)C(=O)[O-].C1=CC=NC(=C1)C(=O)[O-].[Ag] | |
Source | PubChem | |
URL | https://pubchem.ncbi.nlm.nih.gov | |
Description | Data deposited in or computed by PubChem | |
Molecular Formula |
C12H8AgN2O4-2 | |
Source | PubChem | |
URL | https://pubchem.ncbi.nlm.nih.gov | |
Description | Data deposited in or computed by PubChem | |
DSSTOX Substance ID |
DTXSID70552021 | |
Record name | pyridine-2-carboxylate;silver | |
Source | EPA DSSTox | |
URL | https://comptox.epa.gov/dashboard/DTXSID70552021 | |
Description | DSSTox provides a high quality public chemistry resource for supporting improved predictive toxicology. | |
Molecular Weight |
352.07 g/mol | |
Source | PubChem | |
URL | https://pubchem.ncbi.nlm.nih.gov | |
Description | Data deposited in or computed by PubChem | |
CAS No. |
14783-00-7 | |
Record name | pyridine-2-carboxylate;silver | |
Source | EPA DSSTox | |
URL | https://comptox.epa.gov/dashboard/DTXSID70552021 | |
Description | DSSTox provides a high quality public chemistry resource for supporting improved predictive toxicology. | |
Retrosynthesis Analysis
AI-Powered Synthesis Planning: Our tool employs the Template_relevance Pistachio, Template_relevance Bkms_metabolic, Template_relevance Pistachio_ringbreaker, Template_relevance Reaxys, Template_relevance Reaxys_biocatalysis model, leveraging a vast database of chemical reactions to predict feasible synthetic routes.
One-Step Synthesis Focus: Specifically designed for one-step synthesis, it provides concise and direct routes for your target compounds, streamlining the synthesis process.
Accurate Predictions: Utilizing the extensive PISTACHIO, BKMS_METABOLIC, PISTACHIO_RINGBREAKER, REAXYS, REAXYS_BIOCATALYSIS database, our tool offers high-accuracy predictions, reflecting the latest in chemical research and data.
Strategy Settings
Precursor scoring | Relevance Heuristic |
---|---|
Min. plausibility | 0.01 |
Model | Template_relevance |
Template Set | Pistachio/Bkms_metabolic/Pistachio_ringbreaker/Reaxys/Reaxys_biocatalysis |
Top-N result to add to graph | 6 |
Feasible Synthetic Routes
Disclaimer and Information on In-Vitro Research Products
Please be aware that all articles and product information presented on BenchChem are intended solely for informational purposes. The products available for purchase on BenchChem are specifically designed for in-vitro studies, which are conducted outside of living organisms. In-vitro studies, derived from the Latin term "in glass," involve experiments performed in controlled laboratory settings using cells or tissues. It is important to note that these products are not categorized as medicines or drugs, and they have not received approval from the FDA for the prevention, treatment, or cure of any medical condition, ailment, or disease. We must emphasize that any form of bodily introduction of these products into humans or animals is strictly prohibited by law. It is essential to adhere to these guidelines to ensure compliance with legal and ethical standards in research and experimentation.