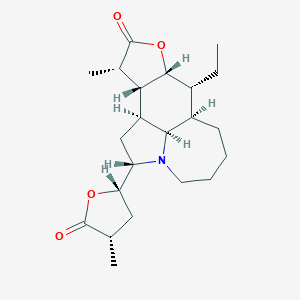
Neotuberostemonine
Overview
Description
Neotuberostemonine is a natural alkaloid isolated from the roots of the plant Stemona tuberosa. This compound is known for its significant biological activities, particularly its antitussive (cough-suppressing) properties. It has been studied for its potential therapeutic effects in various medical conditions, including pulmonary fibrosis and chronic hypoxia-induced lung damage .
Scientific Research Applications
Chemistry: It serves as a model compound for studying the chemical behavior of alkaloids and their derivatives.
Biology: Neotuberostemonine has shown significant biological activities, including antitussive, anti-inflammatory, and antifibrotic effects.
Medicine: It has potential therapeutic applications in treating pulmonary fibrosis, chronic cough, and other respiratory conditions. .
Mechanism of Action
Target of Action
Neotuberostemonine, a natural alkaloid isolated from Stemona tuberosa, primarily targets Hypoxia-Inducible Factor 1-alpha (HIF-1α), Transforming Growth Factor-beta (TGF-β), and Stromal cell-Derived Factor-1 (SDF-1) secreted by macrophages and fibroblasts . These targets play crucial roles in cellular responses to hypoxia and inflammation, and are involved in the regulation of fibroblast activation and differentiation .
Mode of Action
This compound interacts with its targets by suppressing the expression of HIF-1α, TGF-β, and SDF-1 . This suppression is achieved through the regulation of the Phosphoinositide 3-Kinase (PI3K)-dependent Protein Kinase B (AKT) and Extracellular signal-Regulated Kinase (ERK) pathways . The suppression of these targets leads to the inhibition of fibroblast activation and differentiation .
Biochemical Pathways
The biochemical pathways affected by this compound involve the PI3K-dependent AKT and ERK pathways . These pathways are crucial for cell survival, proliferation, and differentiation. By suppressing TGF-β and SDF-1 via these pathways, this compound inhibits the activation and differentiation of fibroblasts, which are key processes in the development of pulmonary fibrosis .
Pharmacokinetics
It’s known that the compound can be orally administered, suggesting good bioavailability
Result of Action
The molecular and cellular effects of this compound’s action include the suppression of HIF-1α, TGF-β, and SDF-1 expression, leading to the inhibition of fibroblast activation and differentiation . In a mouse model of lung fibrosis, this compound effectively attenuated bleomycin-induced pulmonary fibrosis . These results suggest that this compound may be a promising pharmacological agent for the treatment of pulmonary fibrosis .
Action Environment
Hypoxia, or low oxygen levels, is a common condition in many pathological states, including pulmonary fibrosis. This compound’s ability to reverse the effects of hypoxia on lung fibroblasts suggests that its efficacy may be influenced by the oxygen levels in the environment .
Safety and Hazards
Biochemical Analysis
Biochemical Properties
Neotuberostemonine interacts with various enzymes and proteins, playing a significant role in biochemical reactions . It has been found to inhibit the protein expression of Hypoxia-inducible factor 1-alpha (HIF-1α), Transforming growth factor beta (TGF-β), Fibroblast growth factor 2 (FGF2), and Alpha-smooth muscle actin (α-SMA) in hypoxia-exposed fibroblasts .
Cellular Effects
This compound has profound effects on various types of cells and cellular processes . It suppresses the activation and differentiation of primary mouse lung fibroblasts (PLFs) under hypoxic conditions . It also influences cell function by impacting cell signaling pathways, gene expression, and cellular metabolism .
Molecular Mechanism
This compound exerts its effects at the molecular level through several mechanisms . It dose-dependently suppresses hypoxia-induced activation and differentiation of PLFs . It also reverses hypoxia-induced reduction in the expression of prolyl hydroxylated-HIF-1α, a critical step in the initiation of HIF-1α degradation .
Temporal Effects in Laboratory Settings
The effects of this compound change over time in laboratory settings . It has been observed that this compound effectively attenuates bleomycin-induced pulmonary fibrosis in a mouse model when administered orally at a dose of 30 mg·kg -1 ·d -1 for 1 or 2 weeks .
Dosage Effects in Animal Models
The effects of this compound vary with different dosages in animal models . A study found that this compound had a weak inhibitory effect at 15 mg/kg and a strong inhibitory effect at 30 or 60 mg/kg on mouse lung fibrosis .
Metabolic Pathways
It is known to interact with the HIF-1α pathway, influencing the expression of various proteins and enzymes .
Preparation Methods
Synthetic Routes and Reaction Conditions: Neotuberostemonine is primarily obtained through extraction from the roots of Stemona tuberosa. The extraction process involves the use of organic solvents such as methanol or ethanol to isolate the alkaloid from the plant material. The crude extract is then subjected to chromatographic techniques to purify this compound .
Industrial Production Methods: Industrial production of this compound involves large-scale extraction from cultivated Stemona tuberosa plants. The process includes harvesting the roots, drying them, and then using solvent extraction followed by purification steps to obtain the pure compound. The yield and purity of this compound can be optimized by adjusting the extraction parameters and purification techniques .
Chemical Reactions Analysis
Types of Reactions: Neotuberostemonine undergoes various chemical reactions, including:
Oxidation: this compound can be oxidized to form corresponding oxo derivatives.
Reduction: Reduction reactions can convert this compound into its reduced forms.
Substitution: this compound can participate in substitution reactions, where functional groups are replaced by other groups.
Common Reagents and Conditions:
Oxidation: Common oxidizing agents such as potassium permanganate or chromium trioxide can be used.
Reduction: Reducing agents like sodium borohydride or lithium aluminum hydride are typically employed.
Substitution: Various nucleophiles and electrophiles can be used under appropriate conditions to achieve substitution reactions.
Major Products Formed: The major products formed from these reactions depend on the specific reagents and conditions used. For example, oxidation may yield oxo derivatives, while reduction can produce reduced forms of this compound .
Comparison with Similar Compounds
Neotuberostemonine is part of a class of alkaloids known as Stemona alkaloids. Similar compounds include:
Tuberostemonine: Another alkaloid from Stemona tuberosa with similar antitussive and antifibrotic properties.
Neostenine: A derivative of this compound with comparable biological activities.
Uniqueness: this compound is unique due to its specific molecular structure and its ability to inhibit HIF-1α signaling, which is not commonly observed in other related alkaloids. This unique mechanism of action makes it a promising candidate for therapeutic applications in respiratory diseases .
Properties
IUPAC Name |
(1S,3S,9R,10R,11R,14S,15R,16R)-10-ethyl-14-methyl-3-[(2S,4S)-4-methyl-5-oxooxolan-2-yl]-12-oxa-4-azatetracyclo[7.6.1.04,16.011,15]hexadecan-13-one | |
---|---|---|
Source | PubChem | |
URL | https://pubchem.ncbi.nlm.nih.gov | |
Description | Data deposited in or computed by PubChem | |
InChI |
InChI=1S/C22H33NO4/c1-4-13-14-7-5-6-8-23-16(17-9-11(2)21(24)26-17)10-15(19(14)23)18-12(3)22(25)27-20(13)18/h11-20H,4-10H2,1-3H3/t11-,12-,13+,14+,15-,16-,17-,18-,19+,20+/m0/s1 | |
Source | PubChem | |
URL | https://pubchem.ncbi.nlm.nih.gov | |
Description | Data deposited in or computed by PubChem | |
InChI Key |
GYOGHROCTSEKDY-UEIGSNQUSA-N | |
Source | PubChem | |
URL | https://pubchem.ncbi.nlm.nih.gov | |
Description | Data deposited in or computed by PubChem | |
Canonical SMILES |
CCC1C2CCCCN3C2C(CC3C4CC(C(=O)O4)C)C5C1OC(=O)C5C | |
Source | PubChem | |
URL | https://pubchem.ncbi.nlm.nih.gov | |
Description | Data deposited in or computed by PubChem | |
Isomeric SMILES |
CC[C@@H]1[C@H]2CCCCN3[C@H]2[C@@H](C[C@H]3[C@@H]4C[C@@H](C(=O)O4)C)[C@H]5[C@@H]1OC(=O)[C@H]5C | |
Source | PubChem | |
URL | https://pubchem.ncbi.nlm.nih.gov | |
Description | Data deposited in or computed by PubChem | |
Molecular Formula |
C22H33NO4 | |
Source | PubChem | |
URL | https://pubchem.ncbi.nlm.nih.gov | |
Description | Data deposited in or computed by PubChem | |
DSSTOX Substance ID |
DTXSID501316122 | |
Record name | Neotuberostemonine | |
Source | EPA DSSTox | |
URL | https://comptox.epa.gov/dashboard/DTXSID501316122 | |
Description | DSSTox provides a high quality public chemistry resource for supporting improved predictive toxicology. | |
Molecular Weight |
375.5 g/mol | |
Source | PubChem | |
URL | https://pubchem.ncbi.nlm.nih.gov | |
Description | Data deposited in or computed by PubChem | |
CAS No. |
143120-46-1 | |
Record name | Neotuberostemonine | |
Source | CAS Common Chemistry | |
URL | https://commonchemistry.cas.org/detail?cas_rn=143120-46-1 | |
Description | CAS Common Chemistry is an open community resource for accessing chemical information. Nearly 500,000 chemical substances from CAS REGISTRY cover areas of community interest, including common and frequently regulated chemicals, and those relevant to high school and undergraduate chemistry classes. This chemical information, curated by our expert scientists, is provided in alignment with our mission as a division of the American Chemical Society. | |
Explanation | The data from CAS Common Chemistry is provided under a CC-BY-NC 4.0 license, unless otherwise stated. | |
Record name | Neotuberostemonine | |
Source | EPA DSSTox | |
URL | https://comptox.epa.gov/dashboard/DTXSID501316122 | |
Description | DSSTox provides a high quality public chemistry resource for supporting improved predictive toxicology. | |
Retrosynthesis Analysis
AI-Powered Synthesis Planning: Our tool employs the Template_relevance Pistachio, Template_relevance Bkms_metabolic, Template_relevance Pistachio_ringbreaker, Template_relevance Reaxys, Template_relevance Reaxys_biocatalysis model, leveraging a vast database of chemical reactions to predict feasible synthetic routes.
One-Step Synthesis Focus: Specifically designed for one-step synthesis, it provides concise and direct routes for your target compounds, streamlining the synthesis process.
Accurate Predictions: Utilizing the extensive PISTACHIO, BKMS_METABOLIC, PISTACHIO_RINGBREAKER, REAXYS, REAXYS_BIOCATALYSIS database, our tool offers high-accuracy predictions, reflecting the latest in chemical research and data.
Strategy Settings
Precursor scoring | Relevance Heuristic |
---|---|
Min. plausibility | 0.01 |
Model | Template_relevance |
Template Set | Pistachio/Bkms_metabolic/Pistachio_ringbreaker/Reaxys/Reaxys_biocatalysis |
Top-N result to add to graph | 6 |
Feasible Synthetic Routes
Q1: What is neotuberostemonine?
A1: this compound is a bioactive alkaloid primarily found in the roots of Stemona species, particularly Stemona tuberosa. These plants are traditionally used in Asian countries for their antitussive and insecticidal properties. [, ]
Q2: What is the molecular formula and weight of this compound?
A2: The molecular formula of this compound is C22H31NO4, and its molecular weight is 373.49 g/mol. []
Q3: What are the main structural features of this compound?
A3: this compound belongs to the stenine-type of Stemona alkaloids, characterized by a complex tetracyclic pyrrolo[3,2,1-jk][1]benzazepine core structure. [, ]
Q4: How does this compound exert its biological effects?
A4: While the precise mechanisms are still being elucidated, research indicates that this compound may exert its effects through multiple pathways. For instance, it has been shown to:
- Suppress inflammatory responses: this compound can inhibit lipopolysaccharide-induced nitric oxide production in BV2 microglial cells, suggesting anti-inflammatory activity. []
- Modulate macrophage activity: Studies show that it can attenuate bleomycin-induced pulmonary fibrosis, potentially by suppressing macrophage recruitment and activation. [, ]
- Inhibit osteoclastogenesis: Research indicates it can inhibit osteoclast formation by blocking the NF-κB pathway. []
- Reduce cough reflex: this compound demonstrates significant antitussive activity in guinea pig models, potentially by interacting with the saturated tricyclic pyrrolo[3,2,1-jk] benzazepine nucleus. []
Q5: What are the potential therapeutic applications of this compound being investigated?
A5: Based on its observed biological activities, this compound is being explored for its potential in treating:
- Pulmonary fibrosis: Preclinical studies indicate its ability to ameliorate pulmonary fibrosis by suppressing TGF-β and SDF-1 secreted by macrophages and fibroblasts. [, ]
- Inflammatory diseases: Its anti-inflammatory properties make it a potential candidate for conditions involving excessive inflammation. []
- Osteoporosis: The inhibition of osteoclastogenesis suggests a possible role in managing osteoporosis. []
- Cough: Its established antitussive effects point towards its potential as a cough suppressant. []
Q6: What analytical techniques are used to identify and quantify this compound?
A6: Various analytical methods are employed for this compound characterization and quantification, including:
- High-performance liquid chromatography (HPLC): Coupled with diode array detection (DAD) and evaporative light scattering detection (ELSD), HPLC offers a robust method for simultaneous quantification of this compound and other alkaloids in Stemona extracts. [, ]
- Thin-layer chromatography (TLC): TLC combined with image analysis enables the detection and quantification of this compound in plant extracts. []
- Mass spectrometry (MS): Techniques like ultra-performance liquid chromatography-tandem mass spectrometry (UPLC-MS/MS) are valuable for pharmacokinetic studies and metabolic profiling of this compound in biological samples. [, ]
- Nuclear Magnetic Resonance (NMR): NMR spectroscopy, particularly 1D and 2D NMR, plays a crucial role in elucidating the structure and stereochemistry of this compound and its derivatives. [, , , ]
Q7: What is known about the pharmacokinetics of this compound?
A7: Studies in rats using UPLC-MS/MS have provided insights into the absorption, distribution, metabolism, and excretion (ADME) of this compound after oral administration of Stemonae Radix. []
Disclaimer and Information on In-Vitro Research Products
Please be aware that all articles and product information presented on BenchChem are intended solely for informational purposes. The products available for purchase on BenchChem are specifically designed for in-vitro studies, which are conducted outside of living organisms. In-vitro studies, derived from the Latin term "in glass," involve experiments performed in controlled laboratory settings using cells or tissues. It is important to note that these products are not categorized as medicines or drugs, and they have not received approval from the FDA for the prevention, treatment, or cure of any medical condition, ailment, or disease. We must emphasize that any form of bodily introduction of these products into humans or animals is strictly prohibited by law. It is essential to adhere to these guidelines to ensure compliance with legal and ethical standards in research and experimentation.