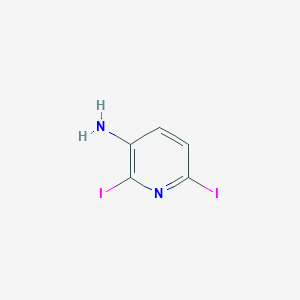
2,6-Diiodopyridin-3-amine
- Click on QUICK INQUIRY to receive a quote from our team of experts.
- With the quality product at a COMPETITIVE price, you can focus more on your research.
Overview
Description
2,6-Diiodopyridin-3-amine is an organic compound that belongs to the class of halogenated pyridines It is characterized by the presence of two iodine atoms at the 2 and 6 positions and an amino group at the 3 position on the pyridine ring
Mechanism of Action
Target of Action
Similar compounds such as 4,6-diarylpyrimidin-2-amine derivatives have shown anticancer properties These compounds may interact with various cellular targets, including enzymes and receptors, to exert their effects
Mode of Action
For instance, some pyrimidine derivatives inhibit enzyme activity, leading to changes in cellular processes
Biochemical Pathways
For example, some pyrimidine derivatives have been shown to affect pathways related to cell growth and proliferation
Pharmacokinetics
Similar compounds such as dopamine receptor agonists have been studied extensively These studies could provide insights into the potential pharmacokinetic properties of 2,6-Diiodopyridin-3-amine
Result of Action
Similar compounds have shown anticancer properties, suggesting that this compound may also have potential anticancer effects
Action Environment
Factors such as ph, temperature, and the presence of other molecules can influence the action of similar compounds
Preparation Methods
Synthetic Routes and Reaction Conditions
The synthesis of 2,6-Diiodopyridin-3-amine typically involves the iodination of pyridine derivatives. One common method is the direct iodination of 3-aminopyridine using iodine and an oxidizing agent such as hydrogen peroxide or sodium hypochlorite. The reaction is usually carried out in an acidic medium to facilitate the iodination process. The general reaction scheme is as follows:
[ \text{3-Aminopyridine} + 2 \text{I}_2 + \text{Oxidizing Agent} \rightarrow \text{this compound} ]
Industrial Production Methods
Industrial production of this compound may involve more efficient and scalable methods, such as continuous flow iodination processes. These methods allow for better control over reaction conditions, higher yields, and reduced production costs. The use of automated systems and advanced purification techniques ensures the consistent quality of the final product.
Chemical Reactions Analysis
Types of Reactions
2,6-Diiodopyridin-3-amine undergoes various chemical reactions, including:
Substitution Reactions: The iodine atoms can be replaced by other substituents through nucleophilic substitution reactions. Common reagents for these reactions include organometallic compounds and halide salts.
Coupling Reactions: The compound can participate in Suzuki-Miyaura and Heck coupling reactions to form biaryl and aryl-alkene derivatives. These reactions typically require palladium catalysts and base conditions.
Reduction Reactions: The iodine atoms can be reduced to form 2,6-diaminopyridine derivatives using reducing agents such as sodium borohydride or lithium aluminum hydride.
Common Reagents and Conditions
Nucleophilic Substitution: Organometallic reagents (e.g., Grignard reagents), halide salts, and polar aprotic solvents (e.g., dimethylformamide).
Coupling Reactions: Palladium catalysts (e.g., Pd(PPh3)4), bases (e.g., potassium carbonate), and solvents (e.g., toluene, ethanol).
Reduction Reactions: Reducing agents (e.g., sodium borohydride, lithium aluminum hydride), solvents (e.g., ethanol, tetrahydrofuran).
Major Products
Substitution Products: Various substituted pyridines depending on the nucleophile used.
Coupling Products: Biaryl and aryl-alkene derivatives.
Reduction Products: 2,6-Diaminopyridine derivatives.
Scientific Research Applications
2,6-Diiodopyridin-3-amine has several applications in scientific research:
Medicinal Chemistry: It serves as a building block for the synthesis of pharmaceutical compounds with potential therapeutic properties, including anticancer and antiviral agents.
Materials Science: The compound is used in the development of advanced materials, such as organic semiconductors and light-emitting diodes (LEDs).
Chemical Biology: It is employed in the design of molecular probes and bioactive molecules for studying biological processes and pathways.
Catalysis: The compound is utilized as a ligand in catalytic reactions, enhancing the efficiency and selectivity of various chemical transformations.
Comparison with Similar Compounds
Similar Compounds
2,6-Dibromopyridin-3-amine: Similar structure with bromine atoms instead of iodine. It exhibits different reactivity and properties due to the smaller size and lower electronegativity of bromine.
2,6-Dichloropyridin-3-amine: Contains chlorine atoms, leading to distinct chemical behavior and applications compared to the iodine derivative.
2,6-Difluoropyridin-3-amine: Fluorine atoms impart unique electronic properties, making it useful in different contexts.
Uniqueness
2,6-Diiodopyridin-3-amine is unique due to the presence of iodine atoms, which confer specific reactivity and properties. The larger atomic size and higher electronegativity of iodine compared to other halogens result in distinct chemical behavior, making this compound valuable for specialized applications in various fields.
Properties
IUPAC Name |
2,6-diiodopyridin-3-amine |
Source
|
---|---|---|
Source | PubChem | |
URL | https://pubchem.ncbi.nlm.nih.gov | |
Description | Data deposited in or computed by PubChem | |
InChI |
InChI=1S/C5H4I2N2/c6-4-2-1-3(8)5(7)9-4/h1-2H,8H2 |
Source
|
Source | PubChem | |
URL | https://pubchem.ncbi.nlm.nih.gov | |
Description | Data deposited in or computed by PubChem | |
InChI Key |
SJSRBFDEPMZNCK-UHFFFAOYSA-N |
Source
|
Source | PubChem | |
URL | https://pubchem.ncbi.nlm.nih.gov | |
Description | Data deposited in or computed by PubChem | |
Canonical SMILES |
C1=CC(=NC(=C1N)I)I |
Source
|
Source | PubChem | |
URL | https://pubchem.ncbi.nlm.nih.gov | |
Description | Data deposited in or computed by PubChem | |
Molecular Formula |
C5H4I2N2 |
Source
|
Source | PubChem | |
URL | https://pubchem.ncbi.nlm.nih.gov | |
Description | Data deposited in or computed by PubChem | |
DSSTOX Substance ID |
DTXSID90400297 |
Source
|
Record name | 2,6-diiodopyridin-3-amine | |
Source | EPA DSSTox | |
URL | https://comptox.epa.gov/dashboard/DTXSID90400297 | |
Description | DSSTox provides a high quality public chemistry resource for supporting improved predictive toxicology. | |
Molecular Weight |
345.91 g/mol |
Source
|
Source | PubChem | |
URL | https://pubchem.ncbi.nlm.nih.gov | |
Description | Data deposited in or computed by PubChem | |
CAS No. |
383131-50-8 |
Source
|
Record name | 2,6-diiodopyridin-3-amine | |
Source | EPA DSSTox | |
URL | https://comptox.epa.gov/dashboard/DTXSID90400297 | |
Description | DSSTox provides a high quality public chemistry resource for supporting improved predictive toxicology. | |
Disclaimer and Information on In-Vitro Research Products
Please be aware that all articles and product information presented on BenchChem are intended solely for informational purposes. The products available for purchase on BenchChem are specifically designed for in-vitro studies, which are conducted outside of living organisms. In-vitro studies, derived from the Latin term "in glass," involve experiments performed in controlled laboratory settings using cells or tissues. It is important to note that these products are not categorized as medicines or drugs, and they have not received approval from the FDA for the prevention, treatment, or cure of any medical condition, ailment, or disease. We must emphasize that any form of bodily introduction of these products into humans or animals is strictly prohibited by law. It is essential to adhere to these guidelines to ensure compliance with legal and ethical standards in research and experimentation.