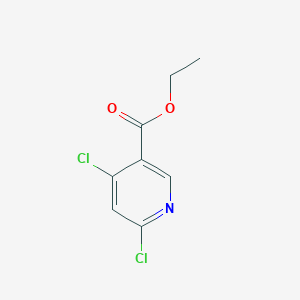
Ethyl 4,6-dichloronicotinate
Overview
Description
Ethyl 4,6-dichloronicotinate is an organic compound with the molecular formula C8H7Cl2NO2. It is a derivative of nicotinic acid, where the hydrogen atoms at positions 4 and 6 on the pyridine ring are replaced by chlorine atoms, and the carboxyl group is esterified with ethanol. This compound is known for its applications in organic synthesis, pharmaceuticals, agrochemicals, and dyestuffs .
Mechanism of Action
Target of Action
Ethyl 4,6-dichloronicotinate is a biochemical reagent that can be used as a biological material or organic compound for life science related research . .
Mode of Action
It is known to act as a reactant in the preparation of (acylamino)aminonaphthyridinones as novobiocin analogs and Hsp90 inhibitors . These compounds have shown antitumor activities in human breast cancer cell lines .
Biochemical Pathways
It is involved in the synthesis of novobiocin analogs and hsp90 inhibitors , which suggests it may influence pathways related to tumor growth and proliferation.
Pharmacokinetics
This compound has a molecular weight of 220.05 . It is soluble in methanol , which suggests it could be well-absorbed in the gastrointestinal tract if administered orally.
Result of Action
It is used in the synthesis of compounds that have shown antitumor activities in human breast cancer cell lines . These compounds have been observed to affect apoptosis and cell cycle advancement .
Action Environment
It is recommended to be stored at room temperature, sealed, and away from moisture , suggesting that its stability could be affected by temperature and humidity.
Preparation Methods
Synthetic Routes and Reaction Conditions: Ethyl 4,6-dichloronicotinate is typically synthesized through the esterification of 4,6-dichloronicotinic acid with ethanol. The reaction is catalyzed by an acid, such as sulfuric acid or hydrochloric acid, under reflux conditions. The general reaction scheme is as follows:
4,6-Dichloronicotinic acid+EthanolAcid catalystEthyl 4,6-dichloronicotinate+Water
Industrial Production Methods: In industrial settings, the synthesis of this compound involves similar esterification processes but on a larger scale. The reaction is carried out in large reactors with efficient mixing and temperature control to ensure high yield and purity. The product is then purified through distillation or recrystallization .
Types of Reactions:
Substitution Reactions: this compound can undergo nucleophilic substitution reactions where the chlorine atoms are replaced by other nucleophiles such as amines or thiols.
Reduction Reactions: The compound can be reduced to form derivatives with different functional groups.
Oxidation Reactions: It can also undergo oxidation to form more complex structures.
Common Reagents and Conditions:
Nucleophilic Substitution: Reagents such as sodium hydroxide or potassium carbonate in polar solvents like dimethyl sulfoxide (DMSO) are commonly used.
Reduction: Catalysts like palladium on carbon (Pd/C) and hydrogen gas are used for reduction reactions.
Oxidation: Oxidizing agents like potassium permanganate (KMnO4) or chromium trioxide (CrO3) are employed.
Major Products:
Substitution Products: Various substituted nicotinates depending on the nucleophile used.
Reduction Products: Reduced forms of the ester, such as alcohols or amines.
Oxidation Products: Oxidized derivatives with additional functional groups.
Scientific Research Applications
Ethyl 4,6-dichloronicotinate is widely used in scientific research due to its versatility:
Chemistry: It serves as a building block in the synthesis of more complex organic molecules.
Biology: The compound is used in the study of enzyme interactions and metabolic pathways.
Medicine: It is a precursor in the synthesis of pharmaceutical compounds, including potential anticancer agents.
Industry: It is used in the production of agrochemicals and dyestuffs
Comparison with Similar Compounds
Ethyl 4,6-dichloronicotinate can be compared with other chlorinated nicotinates, such as:
- Ethyl 3,5-dichloronicotinate
- Ethyl 2,4-dichloronicotinate
- Ethyl 3,6-dichloronicotinate
Uniqueness: The unique positioning of chlorine atoms at the 4 and 6 positions on the pyridine ring of this compound imparts distinct chemical properties, such as increased reactivity and specific biological activity. This makes it particularly useful in the synthesis of specialized pharmaceutical and agrochemical compounds .
Properties
IUPAC Name |
ethyl 4,6-dichloropyridine-3-carboxylate | |
---|---|---|
Source | PubChem | |
URL | https://pubchem.ncbi.nlm.nih.gov | |
Description | Data deposited in or computed by PubChem | |
InChI |
InChI=1S/C8H7Cl2NO2/c1-2-13-8(12)5-4-11-7(10)3-6(5)9/h3-4H,2H2,1H3 | |
Source | PubChem | |
URL | https://pubchem.ncbi.nlm.nih.gov | |
Description | Data deposited in or computed by PubChem | |
InChI Key |
AAUBVINEXCCXOK-UHFFFAOYSA-N | |
Source | PubChem | |
URL | https://pubchem.ncbi.nlm.nih.gov | |
Description | Data deposited in or computed by PubChem | |
Canonical SMILES |
CCOC(=O)C1=CN=C(C=C1Cl)Cl | |
Source | PubChem | |
URL | https://pubchem.ncbi.nlm.nih.gov | |
Description | Data deposited in or computed by PubChem | |
Molecular Formula |
C8H7Cl2NO2 | |
Source | PubChem | |
URL | https://pubchem.ncbi.nlm.nih.gov | |
Description | Data deposited in or computed by PubChem | |
DSSTOX Substance ID |
DTXSID40344874 | |
Record name | Ethyl 4,6-dichloronicotinate | |
Source | EPA DSSTox | |
URL | https://comptox.epa.gov/dashboard/DTXSID40344874 | |
Description | DSSTox provides a high quality public chemistry resource for supporting improved predictive toxicology. | |
Molecular Weight |
220.05 g/mol | |
Source | PubChem | |
URL | https://pubchem.ncbi.nlm.nih.gov | |
Description | Data deposited in or computed by PubChem | |
CAS No. |
40296-46-6 | |
Record name | Ethyl 4,6-dichloronicotinate | |
Source | EPA DSSTox | |
URL | https://comptox.epa.gov/dashboard/DTXSID40344874 | |
Description | DSSTox provides a high quality public chemistry resource for supporting improved predictive toxicology. | |
Record name | Ethyl 4,6-Dichloronicotinate | |
Source | European Chemicals Agency (ECHA) | |
URL | https://echa.europa.eu/information-on-chemicals | |
Description | The European Chemicals Agency (ECHA) is an agency of the European Union which is the driving force among regulatory authorities in implementing the EU's groundbreaking chemicals legislation for the benefit of human health and the environment as well as for innovation and competitiveness. | |
Explanation | Use of the information, documents and data from the ECHA website is subject to the terms and conditions of this Legal Notice, and subject to other binding limitations provided for under applicable law, the information, documents and data made available on the ECHA website may be reproduced, distributed and/or used, totally or in part, for non-commercial purposes provided that ECHA is acknowledged as the source: "Source: European Chemicals Agency, http://echa.europa.eu/". Such acknowledgement must be included in each copy of the material. ECHA permits and encourages organisations and individuals to create links to the ECHA website under the following cumulative conditions: Links can only be made to webpages that provide a link to the Legal Notice page. | |
Synthesis routes and methods
Procedure details
Retrosynthesis Analysis
AI-Powered Synthesis Planning: Our tool employs the Template_relevance Pistachio, Template_relevance Bkms_metabolic, Template_relevance Pistachio_ringbreaker, Template_relevance Reaxys, Template_relevance Reaxys_biocatalysis model, leveraging a vast database of chemical reactions to predict feasible synthetic routes.
One-Step Synthesis Focus: Specifically designed for one-step synthesis, it provides concise and direct routes for your target compounds, streamlining the synthesis process.
Accurate Predictions: Utilizing the extensive PISTACHIO, BKMS_METABOLIC, PISTACHIO_RINGBREAKER, REAXYS, REAXYS_BIOCATALYSIS database, our tool offers high-accuracy predictions, reflecting the latest in chemical research and data.
Strategy Settings
Precursor scoring | Relevance Heuristic |
---|---|
Min. plausibility | 0.01 |
Model | Template_relevance |
Template Set | Pistachio/Bkms_metabolic/Pistachio_ringbreaker/Reaxys/Reaxys_biocatalysis |
Top-N result to add to graph | 6 |
Feasible Synthetic Routes
Q1: Why is Ethyl 4,6-dichloronicotinate a useful starting material for the synthesis of 6-chlorothieno[3,2-c]pyridine-2-carbohydrazide-hydrazone derivatives?
A1: this compound serves as a versatile precursor due to its chemical structure. The molecule contains a pyridine ring with two chlorine atoms and an ethoxycarbonyl group. This allows for a multi-step synthetic route [] involving:
Disclaimer and Information on In-Vitro Research Products
Please be aware that all articles and product information presented on BenchChem are intended solely for informational purposes. The products available for purchase on BenchChem are specifically designed for in-vitro studies, which are conducted outside of living organisms. In-vitro studies, derived from the Latin term "in glass," involve experiments performed in controlled laboratory settings using cells or tissues. It is important to note that these products are not categorized as medicines or drugs, and they have not received approval from the FDA for the prevention, treatment, or cure of any medical condition, ailment, or disease. We must emphasize that any form of bodily introduction of these products into humans or animals is strictly prohibited by law. It is essential to adhere to these guidelines to ensure compliance with legal and ethical standards in research and experimentation.