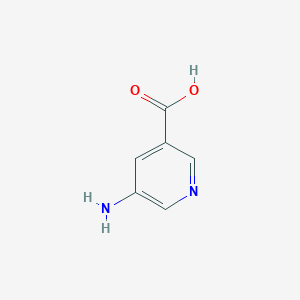
5-Aminonicotinic acid
Overview
Description
5-Aminonicotinic acid is an organic compound with the molecular formula C6H6N2O2. It is a derivative of nicotinic acid, where the amino group is situated at the 5-position of the pyridine ring . This compound is known for its role as a metabolite and its applications in various scientific fields.
Mechanism of Action
Target of Action
5-Aminonicotinic acid, an organic compound with the molecular formula C6H6N2O2 , primarily targets α-amylase and α-glucosidase enzymes . These enzymes play a crucial role in the breakdown of starch into sugars, which is a key process in carbohydrate digestion .
Mode of Action
The synthesized derivatives of this compound have been found to exhibit promising inhibitory activities against α-amylase and α-glucosidase . This means that these compounds can bind to these enzymes and reduce their activity, thereby slowing down the breakdown of starch into sugars .
Biochemical Pathways
The inhibition of α-amylase and α-glucosidase enzymes by this compound derivatives affects the carbohydrate digestion pathway . By slowing down the breakdown of starch into sugars, these compounds can help regulate blood sugar levels, which is particularly beneficial for individuals with diabetes .
Result of Action
The primary result of the action of this compound derivatives is the inhibition of α-amylase and α-glucosidase activities . This leads to a slower breakdown of starch into sugars, which can help regulate blood sugar levels . This makes these compounds potentially useful in the management of diabetes .
Biochemical Analysis
Molecular Mechanism
It is likely that it interacts with biomolecules and influences gene expression, similar to other pyridine derivatives
Metabolic Pathways
5-Aminonicotinic acid is likely involved in various metabolic pathways, given its structural similarity to other pyridine derivatives involved in metabolism
Preparation Methods
Synthetic Routes and Reaction Conditions
5-Aminonicotinic acid can be synthesized through several methods. One common method involves the reaction of 5-bromonicotinic acid with ammonia in the presence of copper sulfate pentahydrate under high pressure and temperature conditions . Another method involves the reaction of this compound with thionyl chloride in methanol, followed by treatment with sodium carbonate to obtain the desired product .
Industrial Production Methods
In industrial settings, the synthesis of this compound often involves the use of large-scale reactors and optimized reaction conditions to ensure high yield and purity. The process typically includes steps such as solvent extraction, crystallization, and purification to obtain the final product.
Chemical Reactions Analysis
Types of Reactions
5-Aminonicotinic acid undergoes various chemical reactions, including:
Oxidation: The amino group can be oxidized to form nitro derivatives.
Reduction: The carboxylic acid group can be reduced to form alcohol derivatives.
Substitution: The amino group can participate in nucleophilic substitution reactions.
Common Reagents and Conditions
Oxidation: Common oxidizing agents include potassium permanganate and hydrogen peroxide.
Reduction: Reducing agents such as lithium aluminum hydride and sodium borohydride are used.
Substitution: Reagents like alkyl halides and acyl chlorides are commonly used in substitution reactions.
Major Products Formed
Oxidation: Nitro-5-nicotinic acid derivatives.
Reduction: 5-Aminonicotinic alcohol derivatives.
Substitution: Various substituted this compound derivatives depending on the reagents used.
Scientific Research Applications
5-Aminonicotinic acid has a wide range of applications in scientific research:
Chemistry: It is used as a building block in the synthesis of various organic compounds and as a ligand in coordination chemistry.
Industry: It is used as an intermediate in the production of pharmaceuticals and agrochemicals.
Comparison with Similar Compounds
Similar Compounds
Nicotinic acid:
Methyl 5-aminonicotinate: A methyl ester derivative of 5-aminonicotinic acid with similar chemical properties.
5-Aminopyridine-3-carboxylic acid: Another derivative with similar functional groups and reactivity.
Uniqueness
This compound is unique due to the presence of both an amino group and a carboxylic acid group on the pyridine ring, which allows it to participate in a wide range of chemical reactions. Its ability to act as both a Bronsted acid and base adds to its versatility in various applications .
Properties
IUPAC Name |
5-aminopyridine-3-carboxylic acid | |
---|---|---|
Source | PubChem | |
URL | https://pubchem.ncbi.nlm.nih.gov | |
Description | Data deposited in or computed by PubChem | |
InChI |
InChI=1S/C6H6N2O2/c7-5-1-4(6(9)10)2-8-3-5/h1-3H,7H2,(H,9,10) | |
Source | PubChem | |
URL | https://pubchem.ncbi.nlm.nih.gov | |
Description | Data deposited in or computed by PubChem | |
InChI Key |
BYIORJAACCWFPU-UHFFFAOYSA-N | |
Source | PubChem | |
URL | https://pubchem.ncbi.nlm.nih.gov | |
Description | Data deposited in or computed by PubChem | |
Canonical SMILES |
C1=C(C=NC=C1N)C(=O)O | |
Source | PubChem | |
URL | https://pubchem.ncbi.nlm.nih.gov | |
Description | Data deposited in or computed by PubChem | |
Molecular Formula |
C6H6N2O2 | |
Source | PubChem | |
URL | https://pubchem.ncbi.nlm.nih.gov | |
Description | Data deposited in or computed by PubChem | |
DSSTOX Substance ID |
DTXSID70326623 | |
Record name | 5-Aminonicotinic acid | |
Source | EPA DSSTox | |
URL | https://comptox.epa.gov/dashboard/DTXSID70326623 | |
Description | DSSTox provides a high quality public chemistry resource for supporting improved predictive toxicology. | |
Molecular Weight |
138.12 g/mol | |
Source | PubChem | |
URL | https://pubchem.ncbi.nlm.nih.gov | |
Description | Data deposited in or computed by PubChem | |
Solubility |
>20.7 [ug/mL] (The mean of the results at pH 7.4) | |
Record name | SID26671297 | |
Source | Burnham Center for Chemical Genomics | |
URL | https://pubchem.ncbi.nlm.nih.gov/bioassay/1996#section=Data-Table | |
Description | Aqueous solubility in buffer at pH 7.4 | |
CAS No. |
24242-19-1 | |
Record name | 5-Aminonicotinic acid | |
Source | EPA DSSTox | |
URL | https://comptox.epa.gov/dashboard/DTXSID70326623 | |
Description | DSSTox provides a high quality public chemistry resource for supporting improved predictive toxicology. | |
Record name | 5-Aminonicotinic Acid | |
Source | European Chemicals Agency (ECHA) | |
URL | https://echa.europa.eu/information-on-chemicals | |
Description | The European Chemicals Agency (ECHA) is an agency of the European Union which is the driving force among regulatory authorities in implementing the EU's groundbreaking chemicals legislation for the benefit of human health and the environment as well as for innovation and competitiveness. | |
Explanation | Use of the information, documents and data from the ECHA website is subject to the terms and conditions of this Legal Notice, and subject to other binding limitations provided for under applicable law, the information, documents and data made available on the ECHA website may be reproduced, distributed and/or used, totally or in part, for non-commercial purposes provided that ECHA is acknowledged as the source: "Source: European Chemicals Agency, http://echa.europa.eu/". Such acknowledgement must be included in each copy of the material. ECHA permits and encourages organisations and individuals to create links to the ECHA website under the following cumulative conditions: Links can only be made to webpages that provide a link to the Legal Notice page. | |
Retrosynthesis Analysis
AI-Powered Synthesis Planning: Our tool employs the Template_relevance Pistachio, Template_relevance Bkms_metabolic, Template_relevance Pistachio_ringbreaker, Template_relevance Reaxys, Template_relevance Reaxys_biocatalysis model, leveraging a vast database of chemical reactions to predict feasible synthetic routes.
One-Step Synthesis Focus: Specifically designed for one-step synthesis, it provides concise and direct routes for your target compounds, streamlining the synthesis process.
Accurate Predictions: Utilizing the extensive PISTACHIO, BKMS_METABOLIC, PISTACHIO_RINGBREAKER, REAXYS, REAXYS_BIOCATALYSIS database, our tool offers high-accuracy predictions, reflecting the latest in chemical research and data.
Strategy Settings
Precursor scoring | Relevance Heuristic |
---|---|
Min. plausibility | 0.01 |
Model | Template_relevance |
Template Set | Pistachio/Bkms_metabolic/Pistachio_ringbreaker/Reaxys/Reaxys_biocatalysis |
Top-N result to add to graph | 6 |
Feasible Synthetic Routes
Disclaimer and Information on In-Vitro Research Products
Please be aware that all articles and product information presented on BenchChem are intended solely for informational purposes. The products available for purchase on BenchChem are specifically designed for in-vitro studies, which are conducted outside of living organisms. In-vitro studies, derived from the Latin term "in glass," involve experiments performed in controlled laboratory settings using cells or tissues. It is important to note that these products are not categorized as medicines or drugs, and they have not received approval from the FDA for the prevention, treatment, or cure of any medical condition, ailment, or disease. We must emphasize that any form of bodily introduction of these products into humans or animals is strictly prohibited by law. It is essential to adhere to these guidelines to ensure compliance with legal and ethical standards in research and experimentation.