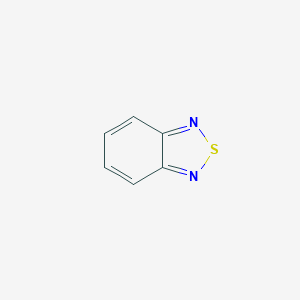
2,1,3-Benzothiadiazole
Overview
Description
2,1,3-Benzothiadiazole is a bicyclic molecule composed of a benzene ring fused to a 1,2,5-thiadiazole ring. This compound has been known since the 19th century and is recognized for its aromatic properties and electron-accepting capabilities . It is widely used in various scientific fields due to its unique chemical structure and properties.
Mechanism of Action
Target of Action
2,1,3-Benzothiadiazole (BT) and its derivatives are primarily used in the development of photoluminescent compounds . They are key acceptor units in the molecular construction of organic light-emitting diodes, organic solar cells, and organic field-effect transistors . Their strong electron-withdrawing ability allows them to improve the electronic properties of the resulting organic materials .
Mode of Action
BT and its derivatives interact with their targets by contributing to the electron transport chain. They are known to undergo standard chemistry of aromatic compounds, readily forming nitro and chloro derivatives . Under reducing conditions, BT can be converted back to the 1,2-diaminobenzene compounds from which they were prepared . This can be a useful way to protect a pair of reactive amino groups while other transformations are performed in the benzene ring to which they are attached .
Biochemical Pathways
The biochemical pathways affected by BT and its derivatives are primarily related to electron transport and energy production. They are used in the development of photoluminescent compounds and are applicable for the molecular construction of organic light-emitting diodes, organic solar cells, and organic field-effect transistors . The exact biochemical pathways and their downstream effects can vary depending on the specific derivative and its application.
Pharmacokinetics
Their strong electron-withdrawing ability and their use in the construction of molecules can usually improve the electronic properties of the resulting organic materials , which may influence their pharmacokinetic properties.
Result of Action
The primary result of BT’s action is the improvement of the electronic properties of the resulting organic materials . This is achieved through their strong electron-withdrawing ability and their role as key acceptor units in the molecular construction of various electronic devices . BT and its derivatives have been found to be luminiscent, with high emission intensity and quantum efficiency .
Biochemical Analysis
Biochemical Properties
2,1,3-Benzothiadiazole and its derivatives are very important acceptor units used in the development of photoluminescent compounds . They are applicable for the molecular construction of organic light-emitting diodes, organic solar cells, and organic field-effect transistors . Due to their strong electron-withdrawing ability, construction of molecules with the unit core of this compound and its derivatives can usually improve the electronic properties of the resulting organic materials .
Cellular Effects
This compound acid derivatives have emerged as versatile new building blocks for the fabrication of smart porous materials .
Molecular Mechanism
Temporal Effects in Laboratory Settings
In solutions, this compound displays a large Stokes-shifted emission as a result of an intramolecular charge-transfer (ICT) reaction in the excited species, followed by a twisting of phenyl moieties . Femtosecond experiments reveal that an ICT reaction takes place in approximately 300 fs while the phenyl twisting occurs in approximately 6 ps .
Preparation Methods
2,1,3-Benzothiadiazole can be synthesized through several methods. One common synthetic route involves the reaction of o-phenylenediamine with two equivalents of thionyl chloride in pyridine, yielding the compound in at least 85% yield . The by-products of this reaction are sulfur dioxide and hydrochloric acid. Another method involves the use of disulfur dichloride, which reacts with anilines to form the intermediate 1,3,2-benzothiazathiolium salt, which is then diazotized to complete the formation of this compound .
Industrial production methods often involve large-scale synthesis using similar reaction conditions but optimized for higher yields and purity. The use of palladium-catalyzed regioselective C-H bond arylations has also been developed for the synthesis of various derivatives of this compound .
Chemical Reactions Analysis
2,1,3-Benzothiadiazole undergoes various chemical reactions, including:
Oxidation and Reduction: Under reducing conditions, 2,1,3-benzothiadiazoles can be converted back to the 1,2-diaminobenzene compounds from which they were prepared.
Substitution Reactions: The compound readily forms nitro and chloro derivatives through standard aromatic substitution reactions.
Functionalization: Regioselective C-H functionalization and aryne reactivity have been explored to create versatile building blocks for further chemical modifications.
Scientific Research Applications
2,1,3-Benzothiadiazole and its derivatives are extensively used in scientific research due to their unique properties:
Comparison with Similar Compounds
2,1,3-Benzothiadiazole is often compared with its isomers, such as 1,2,3-benzothiadiazole and benzo[d][1,2,3]thiadiazole. These compounds share similar structural features but differ in their electronic properties and reactivity:
1,2,3-Benzothiadiazole: This isomer is less nucleophilic and has slower nitration reactions compared to this compound.
Benzo[d][1,2,3]thiadiazole: This compound has higher electron conductivity and stability in the excited state, making it suitable for different applications in organic electronics.
The unique combination of aromaticity, electron-accepting properties, and versatile reactivity makes this compound a valuable compound in various scientific and industrial applications.
Properties
IUPAC Name |
2,1,3-benzothiadiazole | |
---|---|---|
Source | PubChem | |
URL | https://pubchem.ncbi.nlm.nih.gov | |
Description | Data deposited in or computed by PubChem | |
InChI |
InChI=1S/C6H4N2S/c1-2-4-6-5(3-1)7-9-8-6/h1-4H | |
Source | PubChem | |
URL | https://pubchem.ncbi.nlm.nih.gov | |
Description | Data deposited in or computed by PubChem | |
InChI Key |
PDQRQJVPEFGVRK-UHFFFAOYSA-N | |
Source | PubChem | |
URL | https://pubchem.ncbi.nlm.nih.gov | |
Description | Data deposited in or computed by PubChem | |
Canonical SMILES |
C1=CC2=NSN=C2C=C1 | |
Source | PubChem | |
URL | https://pubchem.ncbi.nlm.nih.gov | |
Description | Data deposited in or computed by PubChem | |
Molecular Formula |
C6H4N2S | |
Source | PubChem | |
URL | https://pubchem.ncbi.nlm.nih.gov | |
Description | Data deposited in or computed by PubChem | |
DSSTOX Substance ID |
DTXSID3059767 | |
Record name | 2,1,3-Benzothiadiazole | |
Source | EPA DSSTox | |
URL | https://comptox.epa.gov/dashboard/DTXSID3059767 | |
Description | DSSTox provides a high quality public chemistry resource for supporting improved predictive toxicology. | |
Molecular Weight |
136.18 g/mol | |
Source | PubChem | |
URL | https://pubchem.ncbi.nlm.nih.gov | |
Description | Data deposited in or computed by PubChem | |
CAS No. |
273-13-2 | |
Record name | 2,1,3-Benzothiadiazole | |
Source | CAS Common Chemistry | |
URL | https://commonchemistry.cas.org/detail?cas_rn=273-13-2 | |
Description | CAS Common Chemistry is an open community resource for accessing chemical information. Nearly 500,000 chemical substances from CAS REGISTRY cover areas of community interest, including common and frequently regulated chemicals, and those relevant to high school and undergraduate chemistry classes. This chemical information, curated by our expert scientists, is provided in alignment with our mission as a division of the American Chemical Society. | |
Explanation | The data from CAS Common Chemistry is provided under a CC-BY-NC 4.0 license, unless otherwise stated. | |
Record name | 2,1,3-Benzothiadiazole | |
Source | ChemIDplus | |
URL | https://pubchem.ncbi.nlm.nih.gov/substance/?source=chemidplus&sourceid=0000273132 | |
Description | ChemIDplus is a free, web search system that provides access to the structure and nomenclature authority files used for the identification of chemical substances cited in National Library of Medicine (NLM) databases, including the TOXNET system. | |
Record name | Piazthiole | |
Source | DTP/NCI | |
URL | https://dtp.cancer.gov/dtpstandard/servlet/dwindex?searchtype=NSC&outputformat=html&searchlist=43636 | |
Description | The NCI Development Therapeutics Program (DTP) provides services and resources to the academic and private-sector research communities worldwide to facilitate the discovery and development of new cancer therapeutic agents. | |
Explanation | Unless otherwise indicated, all text within NCI products is free of copyright and may be reused without our permission. Credit the National Cancer Institute as the source. | |
Record name | Piazthiole | |
Source | DTP/NCI | |
URL | https://dtp.cancer.gov/dtpstandard/servlet/dwindex?searchtype=NSC&outputformat=html&searchlist=679 | |
Description | The NCI Development Therapeutics Program (DTP) provides services and resources to the academic and private-sector research communities worldwide to facilitate the discovery and development of new cancer therapeutic agents. | |
Explanation | Unless otherwise indicated, all text within NCI products is free of copyright and may be reused without our permission. Credit the National Cancer Institute as the source. | |
Record name | 2,1,3-Benzothiadiazole | |
Source | EPA Chemicals under the TSCA | |
URL | https://www.epa.gov/chemicals-under-tsca | |
Description | EPA Chemicals under the Toxic Substances Control Act (TSCA) collection contains information on chemicals and their regulations under TSCA, including non-confidential content from the TSCA Chemical Substance Inventory and Chemical Data Reporting. | |
Record name | 2,1,3-Benzothiadiazole | |
Source | EPA DSSTox | |
URL | https://comptox.epa.gov/dashboard/DTXSID3059767 | |
Description | DSSTox provides a high quality public chemistry resource for supporting improved predictive toxicology. | |
Record name | 2,1,3-benzothiadiazole | |
Source | European Chemicals Agency (ECHA) | |
URL | https://echa.europa.eu/substance-information/-/substanceinfo/100.005.442 | |
Description | The European Chemicals Agency (ECHA) is an agency of the European Union which is the driving force among regulatory authorities in implementing the EU's groundbreaking chemicals legislation for the benefit of human health and the environment as well as for innovation and competitiveness. | |
Explanation | Use of the information, documents and data from the ECHA website is subject to the terms and conditions of this Legal Notice, and subject to other binding limitations provided for under applicable law, the information, documents and data made available on the ECHA website may be reproduced, distributed and/or used, totally or in part, for non-commercial purposes provided that ECHA is acknowledged as the source: "Source: European Chemicals Agency, http://echa.europa.eu/". Such acknowledgement must be included in each copy of the material. ECHA permits and encourages organisations and individuals to create links to the ECHA website under the following cumulative conditions: Links can only be made to webpages that provide a link to the Legal Notice page. | |
Record name | 2,1,3-BENZOTHIADIAZOLE | |
Source | FDA Global Substance Registration System (GSRS) | |
URL | https://gsrs.ncats.nih.gov/ginas/app/beta/substances/8FTH8AJA9H | |
Description | The FDA Global Substance Registration System (GSRS) enables the efficient and accurate exchange of information on what substances are in regulated products. Instead of relying on names, which vary across regulatory domains, countries, and regions, the GSRS knowledge base makes it possible for substances to be defined by standardized, scientific descriptions. | |
Explanation | Unless otherwise noted, the contents of the FDA website (www.fda.gov), both text and graphics, are not copyrighted. They are in the public domain and may be republished, reprinted and otherwise used freely by anyone without the need to obtain permission from FDA. Credit to the U.S. Food and Drug Administration as the source is appreciated but not required. | |
Synthesis routes and methods
Procedure details
Retrosynthesis Analysis
AI-Powered Synthesis Planning: Our tool employs the Template_relevance Pistachio, Template_relevance Bkms_metabolic, Template_relevance Pistachio_ringbreaker, Template_relevance Reaxys, Template_relevance Reaxys_biocatalysis model, leveraging a vast database of chemical reactions to predict feasible synthetic routes.
One-Step Synthesis Focus: Specifically designed for one-step synthesis, it provides concise and direct routes for your target compounds, streamlining the synthesis process.
Accurate Predictions: Utilizing the extensive PISTACHIO, BKMS_METABOLIC, PISTACHIO_RINGBREAKER, REAXYS, REAXYS_BIOCATALYSIS database, our tool offers high-accuracy predictions, reflecting the latest in chemical research and data.
Strategy Settings
Precursor scoring | Relevance Heuristic |
---|---|
Min. plausibility | 0.01 |
Model | Template_relevance |
Template Set | Pistachio/Bkms_metabolic/Pistachio_ringbreaker/Reaxys/Reaxys_biocatalysis |
Top-N result to add to graph | 6 |
Feasible Synthetic Routes
Q1: What is the molecular formula and weight of 2,1,3-Benzothiadiazole?
A1: The molecular formula of this compound is C6H4N2S, and its molecular weight is 136.18 g/mol.
Q2: What are the characteristic spectroscopic features of BTD?
A2: BTD derivatives exhibit distinct absorption and emission bands in the UV-Vis and fluorescence spectra, respectively. These bands are sensitive to the nature and position of substituents on the BTD core, allowing for fine-tuning of their optical properties. [, , , , , ]
Q3: How do substituents influence the photophysical properties of BTD derivatives?
A3: Introducing electron-donating or electron-withdrawing substituents at the 4, 5, 6, or 7 positions of the BTD core can significantly alter its electron distribution and conjugation pathway. This results in shifts in absorption and emission wavelengths, enabling the design of BTD-based materials with tailored optical bandgaps and emission colors spanning from blue to red. [, , , , ]
Q4: What is the significance of elastic bending flexibility observed in 4,7-Dibromo-2,1,3-benzothiadiazole crystals?
A4: This property, linked to the specific slip-stacked molecular arrangement in the crystal, is highly desirable for flexible electronic applications. Moreover, the observed reversible fluorescence change upon bending opens avenues for sensing applications. []
Q5: What makes BTD derivatives attractive for organic electronics?
A5: BTD derivatives, particularly when incorporated into conjugated polymers, exhibit valuable properties like high fluorescence quantum yields, tunable energy levels, good charge transport properties, and good thermal stability, making them suitable for applications in organic light-emitting diodes (OLEDs) and organic solar cells (OSCs). [, , , , , , , , , ]
Q6: How does the incorporation of BTD units influence the properties of polyfluorene copolymers?
A6: Incorporating BTD units into polyfluorene backbones introduces intrachain energy transfer pathways, leading to efficient color tuning and enabling the development of single-polymer white-light emitters for OLED applications. [, ]
Q7: What are the advantages of using 2,1,3-naphthothiadiazole (NT) instead of BTD in polymers like PCDTBT for OSCs?
A7: Replacing BTD with NT results in a lower bandgap polymer, leading to a red-shifted absorption profile and potentially improved light-harvesting capabilities in OSCs. Furthermore, the deeper HOMO level in NT-based polymers can contribute to higher open-circuit voltages. []
Q8: What is the significance of the direct arylation polymerization method for synthesizing BTD-based polymers?
A8: This method offers a more cost-effective and environmentally friendly route compared to traditional methods requiring organometallic intermediates, simplifying the synthesis of high-molecular-weight BTD-based polymers for various applications. [, ]
Q9: How does the presence of PCBM ([6,6]-phenyl-C61-butyric acid methyl ester) affect the photostability of BTD-based polymers in OSCs?
A9: The impact of PCBM on photostability is polymer-dependent. While it can offer a stabilizing effect by acting as a light screen or excited state quencher, it can also accelerate degradation in certain cases, highlighting the need for careful material selection and device design considerations. []
Q10: How is computational chemistry employed in understanding the properties of BTD derivatives?
A10: Density Functional Theory (DFT) calculations are valuable for predicting and explaining the electronic structure, optical properties, and redox behavior of BTD derivatives. Time-Dependent DFT (TD-DFT) calculations provide insights into excited state properties, aiding in the design of materials with specific photophysical characteristics. [, , , , , ]
Q11: How does the structure of BTD derivatives relate to their charge transfer properties?
A11: The electron-deficient nature of the BTD core allows it to act as an electron acceptor, enabling the design of donor-acceptor systems. By modifying the donor strength and conjugation pathway through structural variations, the efficiency of charge transfer and its impact on optical and electronic properties can be tuned. [, , , ]
Q12: Can you provide an example of an unprecedented reaction observed during the synthesis of a BTD-based charge transfer complex?
A12: Attempting to create a complex using bis[1,2,5-thiadiazolo][3,4-b;3',4'-e]pyrazine as an acceptor and 4-amino-2,1,3-benzoselenadiazole as a donor resulted in a unique 1:1 addition reaction. This unexpected outcome, involving C-N bond formation and hydrogen atom transfer, highlights the importance of understanding potential reactivity beyond conventional complexation. []
Q13: Are there applications of BTD derivatives beyond organic electronics?
A13: Yes, the unique electronic properties of BTD derivatives make them attractive building blocks for coordination chemistry, leading to the development of novel metal complexes with potential applications in catalysis and sensing. [, ]
Q14: What are the environmental considerations associated with BTD-based materials?
A14: While research is ongoing, understanding the long-term environmental impact and degradation pathways of BTD-based materials is crucial. Developing strategies for recycling, waste management, and exploring biodegradable alternatives will be essential for ensuring their sustainable use. [, ]
Q15: What are the future directions for research on this compound?
A15: Research on BTD derivatives is continuously expanding. Future directions include: - Exploring new synthetic strategies for complex BTD architectures. - Developing structure-property relationships for targeted applications. - Investigating their potential in bioelectronics and bioimaging. - Addressing stability and degradation challenges for long-term device performance. - Evaluating their environmental impact and developing sustainable practices. [, , ]
Disclaimer and Information on In-Vitro Research Products
Please be aware that all articles and product information presented on BenchChem are intended solely for informational purposes. The products available for purchase on BenchChem are specifically designed for in-vitro studies, which are conducted outside of living organisms. In-vitro studies, derived from the Latin term "in glass," involve experiments performed in controlled laboratory settings using cells or tissues. It is important to note that these products are not categorized as medicines or drugs, and they have not received approval from the FDA for the prevention, treatment, or cure of any medical condition, ailment, or disease. We must emphasize that any form of bodily introduction of these products into humans or animals is strictly prohibited by law. It is essential to adhere to these guidelines to ensure compliance with legal and ethical standards in research and experimentation.