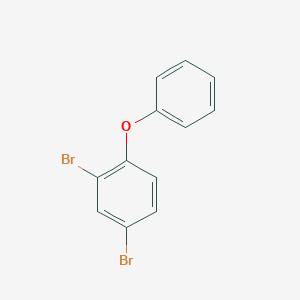
2,4-Dibromodiphenyl ether
Overview
Description
2,4-Dibromodiphenyl ether is a polybromodiphenyl ether that is diphenyl ether in which the hydrogens at the 2 and 4 positions have been replaced by bromines . It is one of the Polybrominated di-Ph ethers (PBDEs), which are a new class of global, persistent, and toxic contaminants .
Synthesis Analysis
The biodegradation of diphenyl ether and transformation of selected brominated congeners by Sphingomonas sp. PH-07 has been studied . This strain mineralized 1 g of diphenyl ether per liter completely within 6 days . The strain PH-07 even catabolized several brominated congeners such as mono-, di-, and tribrominated diphenyl ethers thereby producing the corresponding metabolites .
Molecular Structure Analysis
The molecular formula of this compound is C12H8Br2O . Its average mass is 327.999 Da and its monoisotopic mass is 325.894165 Da .
Chemical Reactions Analysis
The biodegradation of diphenyl ether and transformation of selected brominated congeners by Sphingomonas sp. PH-07 has been studied . This strain mineralized 1 g of diphenyl ether per liter completely within 6 days . The strain PH-07 even catabolized several brominated congeners such as mono-, di-, and tribrominated diphenyl ethers thereby producing the corresponding metabolites .
Physical And Chemical Properties Analysis
The density of this compound is 1.7±0.1 g/cm3 . Its boiling point is 327.9±27.0 °C at 760 mmHg . The vapour pressure is 0.0±0.7 mmHg at 25°C . The enthalpy of vaporization is 54.8±3.0 kJ/mol . The flash point is 132.8±22.2 °C .
Scientific Research Applications
Anaerobic Degradation : Rayne et al. (2003) investigated the anaerobic microbial and photochemical degradation of 4,4'-dibromodiphenyl ether, finding that it was reductively debrominated to produce 4-bromodiphenyl ether and diphenyl ether. This study suggests potential for bioremediation strategies in water treatment processes (Rayne, Ikonomou, & Whale, 2003).
Cosolubilization in Surfactant Systems : Yang et al. (2015) explored the cosolubilization of 4,4′-dibromodiphenyl ether with other compounds in different surfactant micelles. They observed synergism and inhibition in these processes, which could be crucial for understanding the removal of such compounds from contaminated environments (Yang et al., 2015).
Toxicity Studies : Qin et al. (2014) evaluated the toxicity of 4,4′-dibromodiphenyl ether on adult and embryonic zebrafish, indicating moderate toxicity and effects like spine deformation and tail curvature. This research is significant for assessing environmental and health impacts of this compound (Qin et al., 2014).
Photochemical Degradation : Wang et al. (2013) studied the transformation of 2,2',4,4'-tetrabromodiphenyl ether under UV irradiation, finding that it can form less-brominated analogs. This highlights the potential for photolytic degradation as a remediation strategy (Wang et al., 2013).
Biodegradation by Microbial Strains : Schmidt et al. (1993) reported on the biodegradation and transformation of 4,4'-dibromodiphenyl ether by a strain of Sphingomonas, further emphasizing the potential of bioremediation through microbial action (Schmidt, Fortnagel, & Wittich, 1993).
Mechanism of Action
Target of Action
It’s known that certain strains of bacteria, such asCupriavidus sp. WS, can degrade diphenyl ether and its derivatives . The degradation process involves several genes, including bphA1
, bphA2
, and bphA3
.
Mode of Action
The mode of action of 2,4-Dibromodiphenyl ether involves its interaction with the aforementioned genes. The specific roles of bphA
, bphB
, and bphC
were identified by systematically expressing these genes in Escherichia coli. The dihydrodiol product of BphA
was dehydrogenated into 2,3-dihydroxydiphenyl ether by BphB
. 2,3-Dihydroxydiphenyl ether was then decomposed into phenol and 2-pyrone-6-carboxylic acid by BphC
.
Biochemical Pathways
The biochemical pathways affected by this compound involve the sequential action of BphA
, BphB
, and BphC
in the aerobic degradation of diphenyl ether and its derivatives . This degradation process is part of a larger biochemical pathway that helps in the breakdown and removal of persistent environmental pollutants.
Pharmacokinetics
It’s known that the compound has a molecular weight of 327999 , which may influence its bioavailability and pharmacokinetic properties.
Result of Action
The result of the action of this compound is the degradation of the compound into simpler substances. Specifically, this compound is degraded into phenol and 2-pyrone-6-carboxylic acid . This degradation process helps in the removal of this compound from the environment.
Action Environment
The action of this compound is influenced by various environmental factors. For instance, the presence of certain bacteria, such as Cupriavidus sp. WS, is necessary for the degradation of the compound . Additionally, the compound’s stability and efficacy may be affected by factors such as temperature, pH, and the presence of other substances in the environment.
Safety and Hazards
Biochemical Analysis
Biochemical Properties
2,4-Dibromodiphenyl ether plays a significant role in biochemical reactions, particularly in its interactions with enzymes and proteins involved in detoxification processes. It is known to interact with cytochrome P450 enzymes, which are responsible for the oxidative metabolism of various xenobiotics. The interaction between this compound and cytochrome P450 enzymes leads to the formation of reactive metabolites that can bind to cellular macromolecules, potentially causing cellular damage . Additionally, this compound can interact with glutathione S-transferases, which are involved in the conjugation and detoxification of reactive metabolites .
Cellular Effects
This compound has been shown to affect various types of cells and cellular processes. It can disrupt cell signaling pathways, leading to altered gene expression and cellular metabolism. Studies have demonstrated that this compound can induce oxidative stress in cells, resulting in the production of reactive oxygen species (ROS) and subsequent damage to cellular components . This compound has also been found to interfere with the endocrine system by mimicking or antagonizing the actions of natural hormones, thereby affecting hormone-regulated cellular functions .
Molecular Mechanism
The molecular mechanism of action of this compound involves its binding interactions with various biomolecules. It can bind to nuclear receptors, such as the aryl hydrocarbon receptor (AhR) and the estrogen receptor, leading to changes in gene expression . Additionally, this compound can inhibit or activate enzymes involved in metabolic pathways, further influencing cellular functions . The compound’s ability to generate reactive metabolites through cytochrome P450-mediated oxidation also contributes to its toxic effects at the molecular level .
Temporal Effects in Laboratory Settings
In laboratory settings, the effects of this compound can change over time due to its stability and degradation properties. Studies have shown that this compound is relatively stable under environmental conditions, leading to its persistence in biological systems . Over time, the accumulation of this compound and its metabolites can result in long-term effects on cellular function, including chronic oxidative stress and disruption of cellular homeostasis . In vitro and in vivo studies have demonstrated that prolonged exposure to this compound can lead to significant alterations in cellular processes .
Dosage Effects in Animal Models
The effects of this compound vary with different dosages in animal models. At low doses, the compound may induce mild oxidative stress and minor alterations in gene expression . At higher doses, this compound can cause severe toxic effects, including liver damage, neurotoxicity, and endocrine disruption . Threshold effects have been observed, where certain dosages result in significant adverse effects, highlighting the importance of dose-dependent toxicity assessments .
Metabolic Pathways
This compound is involved in several metabolic pathways, primarily through its interactions with cytochrome P450 enzymes and glutathione S-transferases . The oxidative metabolism of this compound by cytochrome P450 enzymes leads to the formation of reactive metabolites, which can be further conjugated by glutathione S-transferases for detoxification . These metabolic processes influence the compound’s bioavailability and toxicity, affecting metabolic flux and metabolite levels in biological systems .
Transport and Distribution
Within cells and tissues, this compound is transported and distributed through various mechanisms. It can interact with transporters and binding proteins that facilitate its movement across cellular membranes . The compound’s lipophilic nature allows it to accumulate in lipid-rich tissues, such as adipose tissue and the liver . This accumulation can lead to prolonged exposure and potential toxic effects in these tissues .
Subcellular Localization
The subcellular localization of this compound can influence its activity and function. Studies have shown that this compound can localize to specific cellular compartments, such as the endoplasmic reticulum and mitochondria . The targeting of this compound to these organelles can affect their functions, including protein synthesis, energy production, and cellular signaling . Post-translational modifications and targeting signals may play a role in directing the compound to specific subcellular locations .
properties
IUPAC Name |
2,4-dibromo-1-phenoxybenzene | |
---|---|---|
Source | PubChem | |
URL | https://pubchem.ncbi.nlm.nih.gov | |
Description | Data deposited in or computed by PubChem | |
InChI |
InChI=1S/C12H8Br2O/c13-9-6-7-12(11(14)8-9)15-10-4-2-1-3-5-10/h1-8H | |
Source | PubChem | |
URL | https://pubchem.ncbi.nlm.nih.gov | |
Description | Data deposited in or computed by PubChem | |
InChI Key |
JMCIHKKTRDLVCO-UHFFFAOYSA-N | |
Source | PubChem | |
URL | https://pubchem.ncbi.nlm.nih.gov | |
Description | Data deposited in or computed by PubChem | |
Canonical SMILES |
C1=CC=C(C=C1)OC2=C(C=C(C=C2)Br)Br | |
Source | PubChem | |
URL | https://pubchem.ncbi.nlm.nih.gov | |
Description | Data deposited in or computed by PubChem | |
Molecular Formula |
C12H8Br2O | |
Source | PubChem | |
URL | https://pubchem.ncbi.nlm.nih.gov | |
Description | Data deposited in or computed by PubChem | |
DSSTOX Substance ID |
DTXSID70565813 | |
Record name | 2,4-Dibromo-1-phenoxybenzene | |
Source | EPA DSSTox | |
URL | https://comptox.epa.gov/dashboard/DTXSID70565813 | |
Description | DSSTox provides a high quality public chemistry resource for supporting improved predictive toxicology. | |
Molecular Weight |
328.00 g/mol | |
Source | PubChem | |
URL | https://pubchem.ncbi.nlm.nih.gov | |
Description | Data deposited in or computed by PubChem | |
CAS RN |
171977-44-9 | |
Record name | 2,4-Dibromodiphenyl ether | |
Source | ChemIDplus | |
URL | https://pubchem.ncbi.nlm.nih.gov/substance/?source=chemidplus&sourceid=0171977449 | |
Description | ChemIDplus is a free, web search system that provides access to the structure and nomenclature authority files used for the identification of chemical substances cited in National Library of Medicine (NLM) databases, including the TOXNET system. | |
Record name | 2,4-Dibromo-1-phenoxybenzene | |
Source | EPA DSSTox | |
URL | https://comptox.epa.gov/dashboard/DTXSID70565813 | |
Description | DSSTox provides a high quality public chemistry resource for supporting improved predictive toxicology. | |
Record name | 2,4-DIBROMODIPHENYL ETHER | |
Source | FDA Global Substance Registration System (GSRS) | |
URL | https://gsrs.ncats.nih.gov/ginas/app/beta/substances/M0M026GK5L | |
Description | The FDA Global Substance Registration System (GSRS) enables the efficient and accurate exchange of information on what substances are in regulated products. Instead of relying on names, which vary across regulatory domains, countries, and regions, the GSRS knowledge base makes it possible for substances to be defined by standardized, scientific descriptions. | |
Explanation | Unless otherwise noted, the contents of the FDA website (www.fda.gov), both text and graphics, are not copyrighted. They are in the public domain and may be republished, reprinted and otherwise used freely by anyone without the need to obtain permission from FDA. Credit to the U.S. Food and Drug Administration as the source is appreciated but not required. | |
Retrosynthesis Analysis
AI-Powered Synthesis Planning: Our tool employs the Template_relevance Pistachio, Template_relevance Bkms_metabolic, Template_relevance Pistachio_ringbreaker, Template_relevance Reaxys, Template_relevance Reaxys_biocatalysis model, leveraging a vast database of chemical reactions to predict feasible synthetic routes.
One-Step Synthesis Focus: Specifically designed for one-step synthesis, it provides concise and direct routes for your target compounds, streamlining the synthesis process.
Accurate Predictions: Utilizing the extensive PISTACHIO, BKMS_METABOLIC, PISTACHIO_RINGBREAKER, REAXYS, REAXYS_BIOCATALYSIS database, our tool offers high-accuracy predictions, reflecting the latest in chemical research and data.
Strategy Settings
Precursor scoring | Relevance Heuristic |
---|---|
Min. plausibility | 0.01 |
Model | Template_relevance |
Template Set | Pistachio/Bkms_metabolic/Pistachio_ringbreaker/Reaxys/Reaxys_biocatalysis |
Top-N result to add to graph | 6 |
Feasible Synthetic Routes
Q & A
Q1: What is the environmental significance of 2,4-Dibromodiphenyl ether?
A: this compound is a polybrominated diphenyl ether (PBDE) congener. PBDEs are a class of flame retardants used in various products, raising concerns about their persistence and potential for bioaccumulation in the environment. Studies have detected this compound in indoor and outdoor environments, including air and water samples. []
Q2: What were the key findings regarding this compound in the indoor air study?
A: The study investigating airborne PBDEs in a Taiwanese college classroom found significantly higher indoor concentrations of this compound compared to outdoor levels. [] This finding suggests indoor sources, potentially computer-related products, contribute to elevated this compound levels in these environments. The authors recommend specific measures like increased ventilation and using products compliant with the Restriction of Hazardous Substances Directive to mitigate indoor exposure. []
Q3: How does the chemical structure of this compound relate to its reactivity with OH radicals?
A: Research on the gas-phase reactions of PBDEs, including this compound, with OH radicals provides insights into their atmospheric fate. The study determined the OH radical rate constant for this compound to be 3.8 x 10^-12 cm3 molecule-1 s-1 at 298 K. [] The study also identified bromophenols and Br2 as products of these reactions, suggesting that OH addition to ipso positions on the aromatic ring is a significant pathway. []
Disclaimer and Information on In-Vitro Research Products
Please be aware that all articles and product information presented on BenchChem are intended solely for informational purposes. The products available for purchase on BenchChem are specifically designed for in-vitro studies, which are conducted outside of living organisms. In-vitro studies, derived from the Latin term "in glass," involve experiments performed in controlled laboratory settings using cells or tissues. It is important to note that these products are not categorized as medicines or drugs, and they have not received approval from the FDA for the prevention, treatment, or cure of any medical condition, ailment, or disease. We must emphasize that any form of bodily introduction of these products into humans or animals is strictly prohibited by law. It is essential to adhere to these guidelines to ensure compliance with legal and ethical standards in research and experimentation.