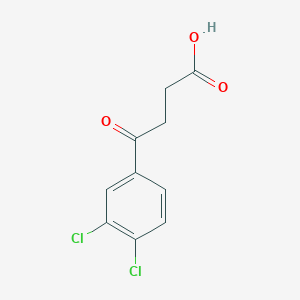
4-(3,4-Dichlorophenyl)-4-oxobutanoic acid
Overview
Description
4-(3,4-Dichlorophenyl)-4-oxobutanoic acid is a substituted butanoic acid derivative featuring a 3,4-dichlorophenyl group attached to a ketone-bearing carbon chain. Its synthesis is typically achieved via Friedel-Crafts acylation, where succinic anhydride reacts with 1,2-dichlorobenzene under optimized conditions (mole ratio 1:6, 60°C, 4 hours), yielding a 94% product . Studies highlight its role as a precursor for kynurenine 3-hydroxylase inhibitors, which are relevant in neurodegenerative disease research .
Preparation Methods
Synthetic Routes and Reaction Conditions
The synthesis of 4-(3,4-Dichlorophenyl)-4-oxobutanoic acid typically involves the reaction of 3,4-dichlorobenzaldehyde with malonic acid in the presence of a base, followed by decarboxylation. Another method involves the reaction of 3,4-dichlorophenylacetic acid with acetic anhydride . The reaction conditions often require refluxing in an appropriate solvent such as benzene or ethanol .
Industrial Production Methods
Industrial production methods for this compound are not extensively documented. the synthesis routes mentioned above can be scaled up for industrial applications, provided that the reaction conditions are optimized for large-scale production.
Chemical Reactions Analysis
Types of Reactions
4-(3,4-Dichlorophenyl)-4-oxobutanoic acid undergoes various chemical reactions, including:
Oxidation: The compound can be oxidized to form corresponding carboxylic acids.
Reduction: Reduction reactions can convert the ketone group to an alcohol.
Substitution: The dichlorophenyl group can undergo nucleophilic substitution reactions.
Common Reagents and Conditions
Oxidation: Common oxidizing agents include potassium permanganate and chromium trioxide.
Reduction: Sodium borohydride and lithium aluminum hydride are typical reducing agents.
Substitution: Nucleophiles such as amines and thiols can be used for substitution reactions.
Major Products
Oxidation: Formation of carboxylic acids.
Reduction: Formation of alcohols.
Substitution: Formation of substituted phenyl derivatives.
Scientific Research Applications
Synthesis of 4-(3,4-Dichlorophenyl)-4-oxobutanoic Acid
The synthesis typically involves multi-step organic reactions, which can be modified based on the desired yield and purity. Key steps may include:
- Formation of the Oxobutanoic Acid Backbone : Utilizing appropriate starting materials to construct the oxobutanoic acid framework.
- Substitution Reactions : Introducing the dichlorophenyl group through electrophilic aromatic substitution.
- Purification : Employing crystallization or chromatography techniques to isolate the final product.
Medicinal Chemistry
The compound is primarily explored for its potential as a pharmaceutical lead in developing drugs targeting neurodegenerative diseases. Its role as an inhibitor of kynurenine 3-hydroxylase links it to pathways involved in conditions such as Alzheimer's disease, Parkinson's disease, and Huntington's chorea .
Table 1: Biological Activities Related to Neurodegenerative Diseases
Biological Interactions
Research indicates that derivatives of this compound exhibit various biological activities, including:
- Antimicrobial properties against both Gram-positive and Gram-negative bacteria.
- Potential anti-inflammatory effects observed in preclinical studies.
Table 2: Antimicrobial Activity of Derivatives
Compound Name | Activity Against | Efficacy Level |
---|---|---|
This compound | Gram-positive bacteria | High |
This compound | Gram-negative bacteria | Moderate |
Industrial Applications
Beyond medicinal uses, this compound can serve as a building block in organic synthesis for creating more complex molecules. Its unique structure allows for versatile applications in developing new materials and chemical products .
Case Studies and Experimental Findings
Several studies have documented the efficacy of this compound in various experimental settings:
- A study demonstrated its inhibitory effects on kynurenine 3-hydroxylase with an IC50 value indicating significant potency .
- Another research project explored its interactions with biological receptors using molecular docking simulations, revealing high binding affinities that suggest potential therapeutic uses .
Mechanism of Action
The mechanism of action of 4-(3,4-Dichlorophenyl)-4-oxobutanoic acid involves its interaction with specific molecular targets. For instance, it can inhibit certain enzymes or receptors, leading to its biological effects. The exact pathways and molecular targets are still under investigation, but it is known to affect cellular processes such as signal transduction and metabolic pathways .
Comparison with Similar Compounds
Comparison with Structurally Similar Compounds
Structural Analogs and Substituent Variations
The biological and physicochemical properties of 4-(3,4-dichlorophenyl)-4-oxobutanoic acid are influenced by substituent modifications on the phenyl ring or the butanoic acid chain. Below is a comparative analysis of key analogs:
Table 1: Structural and Functional Comparison of Analogous Compounds
Impact of Substituents on Activity
- Halogen Substitution : Chlorine atoms at the 3,4-positions enhance electron-withdrawing effects, increasing binding affinity to enzymes like kynurenine 3-hydroxylase. Fluorine analogs (e.g., 3,4-difluoro derivatives) exhibit reduced molecular weight and altered pharmacokinetics due to lower lipophilicity .
- Functional Group Modifications: The addition of a 2-hydroxy group (compound 8) improves inhibitory potency against kynurenine 3-hydroxylase compared to the parent compound . Amino-substituted derivatives (e.g., compound 47) demonstrate antivirulence activity, likely due to enhanced hydrogen bonding with bacterial targets .
- Ring Position and Bulkiness : Propyl or cyclohexyl groups (e.g., 4-propyl-3,5-dichloro derivative) increase steric bulk, enhancing COX-2 selectivity .
Research Findings and Implications
- Enzyme Inhibition : Compound 8 shows 10-fold greater potency than the parent acid in kynurenine 3-hydroxylase inhibition, underscoring the role of hydroxyl groups in active-site interactions .
- Antimicrobial Activity: Heterocyclic derivatives (e.g., pyridazinones) derived from this compound exhibit broad-spectrum antimicrobial effects, with MIC values as low as 8 µg/mL against S. aureus .
Biological Activity
4-(3,4-Dichlorophenyl)-4-oxobutanoic acid, also known as PCPA (Phenyl-4-oxobutanoic acid), is a compound notable for its potential biological activities, particularly in the context of neuroprotection and inflammation. Its structure, characterized by a dichlorophenyl group attached to a 4-oxobutanoic acid moiety, suggests diverse reactivity and interaction capabilities with biological systems.
- Molecular Formula : C16H11Cl3O3
- Molecular Weight : 357.61 g/mol
- Structural Characteristics : The compound contains two stereogenic centers, allowing it to act as a chiral auxiliary in asymmetric synthesis. This property enhances its utility in drug development and synthesis of biologically active compounds.
Biological Activity Overview
Research has indicated that this compound exhibits significant biological activity, particularly as an inhibitor of kynurenine 3-hydroxylase (KMO). This enzyme is crucial in the kynurenine pathway of tryptophan metabolism, which is linked to various neurological disorders.
Kynurenine 3-hydroxylase is involved in the production of neurotoxic metabolites. Inhibition of this enzyme can mitigate excitotoxicity and neuroinflammation, making PCPA a candidate for therapeutic strategies against conditions such as Alzheimer's disease and other neurodegenerative disorders.
In Vitro Studies
Recent in vitro studies have demonstrated that derivatives of PCPA effectively inhibit KMO activity. The structure-activity relationship (SAR) analysis has revealed that modifications to the compound can enhance its inhibitory effects:
Modification | Effect on KMO Inhibition |
---|---|
Methyl group addition | Increased potency |
Hydroxyl substitution | Decreased activity |
Alteration of the phenyl group | Variable effects depending on substituents |
These findings suggest that careful structural modifications can optimize the compound's biological efficacy.
Anti-inflammatory Properties
PCPA has also been evaluated for its anti-inflammatory properties. Studies indicate that it can reduce pro-inflammatory cytokine levels in cell cultures, suggesting potential applications in treating inflammatory diseases.
Case Studies
-
Neuroprotective Effects :
A study conducted by researchers at the University of Ural investigated the neuroprotective effects of PCPA in animal models of neurodegeneration. Results showed significant reduction in neuronal loss and improvement in cognitive functions following treatment with PCPA derivatives. -
Anti-inflammatory Activity :
Research published in Pharmaceutical Chemistry Journal highlighted the anti-inflammatory effects of PCPA analogs. The study reported a decrease in inflammation markers in both in vitro and in vivo models, indicating its potential as an anti-inflammatory agent.
Comparative Analysis with Related Compounds
The following table compares PCPA with structurally similar compounds regarding their biological activities:
Compound Name | Molecular Formula | Key Features | Biological Activity |
---|---|---|---|
7-(3,4-Dichlorophenyl)-7-oxoheptanoic acid | C13H10Cl2O3 | Heptanoic backbone | Anti-inflammatory |
6-(3,4-Dichlorophenyl)-6-oxohexanoic acid | C12H9Cl2O3 | Hexanoic chain | Enzyme inhibition |
8-(3,4-Dichlorophenyl)-8-oxooctanoic acid | C14H12Cl2O3 | Octanoic backbone | Drug design applications |
5-(3,4-Dichlorophenyl)-5-oxovaleric acid | C11H8Cl2O3 | Valeric structure | Biological target interactions |
Q & A
Basic Research Questions
Q. What synthetic methodologies are commonly employed to prepare 4-(3,4-Dichlorophenyl)-4-oxobutanoic acid, and how is purity validated?
- Answer : The compound is typically synthesized via Friedel-Crafts acylation using maleic anhydride and dichlorobenzene derivatives, followed by hydrolysis . Alternative routes include Knoevenagel condensation with substituted benzaldehydes under alkaline conditions, as seen in fluorophenyl analogs . Purity is validated using HPLC (reversed-phase C18 column, acetonitrile/water mobile phase) and corroborated by H/C NMR (e.g., ketone carbonyl resonance at ~200 ppm) and high-resolution mass spectrometry (HRMS) .
Q. How do electron-withdrawing chlorine substituents on the phenyl ring influence the compound’s reactivity in nucleophilic additions?
- Answer : The 3,4-dichloro groups enhance electrophilicity at the ketone via resonance and inductive effects, facilitating nucleophilic attacks (e.g., by hydrazines or amines). This is evident in reactions with antipyrin, where the ketone undergoes condensation to form hydrazone or pyridazinone derivatives . Comparative studies with fluorophenyl analogs show slower reaction kinetics due to fluorine’s weaker electron-withdrawing effect .
Q. What spectroscopic techniques are optimal for characterizing this compound and its derivatives?
- Answer : Key techniques include:
- FT-IR : Confirms ketone (C=O stretch at ~1700 cm) and carboxylic acid (broad O-H stretch at ~2500–3000 cm) functionalities .
- NMR : H NMR identifies aromatic protons (δ 7.4–8.1 ppm, doublets) and α-protons to the ketone (δ 3.1–3.5 ppm). C NMR resolves the carbonyl carbons (ketone at ~200 ppm, carboxylic acid at ~175 ppm) .
- X-ray crystallography : Determines stereochemistry in crystalline derivatives, such as pyridazinones .
Advanced Research Questions
Q. How can the ketone group in this compound be functionalized to synthesize bioactive heterocycles?
- Answer : The ketone undergoes condensation reactions with nucleophiles like antipyrin or phenylhydrazine to form hydrazones, which cyclize under acidic or thermal conditions. For example:
- Reaction with antipyrin in dry benzene yields 4-antipyrinyl derivatives, which further react with hydrazines to form pyridazinones (Scheme 1 in ).
- Michael addition with thioglycolic acid produces sulfanyl derivatives, enhancing solubility for biological assays . Optimized conditions (e.g., ethanol reflux, 72 hours) achieve >80% yields .
Q. What strategies resolve enantiomers in derivatives of this compound?
- Answer : Chiral derivatization using (-)-menthol or (+)-α-methylbenzylamine followed by HPLC (chiral stationary phase, e.g., Chiralpak AD-H) resolves enantiomers. Asymmetric catalysis (e.g., BINOL-phosphoric acid catalysts) directly synthesizes enantiopure pyridazinones, with enantiomeric excess (ee) verified by circular dichroism (CD) .
Q. How do computational methods predict the bioactivity of novel derivatives, and how do they align with experimental data?
- Answer : Docking studies (AutoDock Vina) model interactions with target enzymes (e.g., COX-2 or Kynurenine-3-hydroxylase). QSAR models using Hammett σ constants for substituents correlate logP with antimicrobial IC values. For example, derivatives with electron-withdrawing groups show enhanced binding to COX-2 (ΔG = -9.2 kcal/mol) . Experimental MIC values against S. aureus (2–8 µg/mL) align with predictions .
Q. Data Contradictions and Mitigation
- Synthetic Yields : reports 72-hour reaction times for pyridazinones, while fluorophenyl analogs in require shorter durations (24 hours). Mitigation: Optimize temperature (70°C vs. 25°C) and catalyst loading.
- Bioactivity Variability : Antimicrobial activity varies with substituent position. For example, 3,4-dichloro derivatives outperform 4-chloro analogs in antifungal assays. Use combinatorial libraries to map structure-activity relationships .
Properties
IUPAC Name |
4-(3,4-dichlorophenyl)-4-oxobutanoic acid | |
---|---|---|
Source | PubChem | |
URL | https://pubchem.ncbi.nlm.nih.gov | |
Description | Data deposited in or computed by PubChem | |
InChI |
InChI=1S/C10H8Cl2O3/c11-7-2-1-6(5-8(7)12)9(13)3-4-10(14)15/h1-2,5H,3-4H2,(H,14,15) | |
Source | PubChem | |
URL | https://pubchem.ncbi.nlm.nih.gov | |
Description | Data deposited in or computed by PubChem | |
InChI Key |
DPCXOOJTAKKWIC-UHFFFAOYSA-N | |
Source | PubChem | |
URL | https://pubchem.ncbi.nlm.nih.gov | |
Description | Data deposited in or computed by PubChem | |
Canonical SMILES |
C1=CC(=C(C=C1C(=O)CCC(=O)O)Cl)Cl | |
Source | PubChem | |
URL | https://pubchem.ncbi.nlm.nih.gov | |
Description | Data deposited in or computed by PubChem | |
Molecular Formula |
C10H8Cl2O3 | |
Source | PubChem | |
URL | https://pubchem.ncbi.nlm.nih.gov | |
Description | Data deposited in or computed by PubChem | |
DSSTOX Substance ID |
DTXSID60302592 | |
Record name | 4-(3,4-dichlorophenyl)-4-oxobutanoic acid | |
Source | EPA DSSTox | |
URL | https://comptox.epa.gov/dashboard/DTXSID60302592 | |
Description | DSSTox provides a high quality public chemistry resource for supporting improved predictive toxicology. | |
Molecular Weight |
247.07 g/mol | |
Source | PubChem | |
URL | https://pubchem.ncbi.nlm.nih.gov | |
Description | Data deposited in or computed by PubChem | |
CAS No. |
50597-19-8 | |
Record name | 50597-19-8 | |
Source | DTP/NCI | |
URL | https://dtp.cancer.gov/dtpstandard/servlet/dwindex?searchtype=NSC&outputformat=html&searchlist=152012 | |
Description | The NCI Development Therapeutics Program (DTP) provides services and resources to the academic and private-sector research communities worldwide to facilitate the discovery and development of new cancer therapeutic agents. | |
Explanation | Unless otherwise indicated, all text within NCI products is free of copyright and may be reused without our permission. Credit the National Cancer Institute as the source. | |
Record name | 4-(3,4-dichlorophenyl)-4-oxobutanoic acid | |
Source | EPA DSSTox | |
URL | https://comptox.epa.gov/dashboard/DTXSID60302592 | |
Description | DSSTox provides a high quality public chemistry resource for supporting improved predictive toxicology. | |
Record name | 4-(3,4-Dichlorophenyl)-4-oxobutyric acid | |
Source | European Chemicals Agency (ECHA) | |
URL | https://echa.europa.eu/information-on-chemicals | |
Description | The European Chemicals Agency (ECHA) is an agency of the European Union which is the driving force among regulatory authorities in implementing the EU's groundbreaking chemicals legislation for the benefit of human health and the environment as well as for innovation and competitiveness. | |
Explanation | Use of the information, documents and data from the ECHA website is subject to the terms and conditions of this Legal Notice, and subject to other binding limitations provided for under applicable law, the information, documents and data made available on the ECHA website may be reproduced, distributed and/or used, totally or in part, for non-commercial purposes provided that ECHA is acknowledged as the source: "Source: European Chemicals Agency, http://echa.europa.eu/". Such acknowledgement must be included in each copy of the material. ECHA permits and encourages organisations and individuals to create links to the ECHA website under the following cumulative conditions: Links can only be made to webpages that provide a link to the Legal Notice page. | |
Retrosynthesis Analysis
AI-Powered Synthesis Planning: Our tool employs the Template_relevance Pistachio, Template_relevance Bkms_metabolic, Template_relevance Pistachio_ringbreaker, Template_relevance Reaxys, Template_relevance Reaxys_biocatalysis model, leveraging a vast database of chemical reactions to predict feasible synthetic routes.
One-Step Synthesis Focus: Specifically designed for one-step synthesis, it provides concise and direct routes for your target compounds, streamlining the synthesis process.
Accurate Predictions: Utilizing the extensive PISTACHIO, BKMS_METABOLIC, PISTACHIO_RINGBREAKER, REAXYS, REAXYS_BIOCATALYSIS database, our tool offers high-accuracy predictions, reflecting the latest in chemical research and data.
Strategy Settings
Precursor scoring | Relevance Heuristic |
---|---|
Min. plausibility | 0.01 |
Model | Template_relevance |
Template Set | Pistachio/Bkms_metabolic/Pistachio_ringbreaker/Reaxys/Reaxys_biocatalysis |
Top-N result to add to graph | 6 |
Feasible Synthetic Routes
Disclaimer and Information on In-Vitro Research Products
Please be aware that all articles and product information presented on BenchChem are intended solely for informational purposes. The products available for purchase on BenchChem are specifically designed for in-vitro studies, which are conducted outside of living organisms. In-vitro studies, derived from the Latin term "in glass," involve experiments performed in controlled laboratory settings using cells or tissues. It is important to note that these products are not categorized as medicines or drugs, and they have not received approval from the FDA for the prevention, treatment, or cure of any medical condition, ailment, or disease. We must emphasize that any form of bodily introduction of these products into humans or animals is strictly prohibited by law. It is essential to adhere to these guidelines to ensure compliance with legal and ethical standards in research and experimentation.