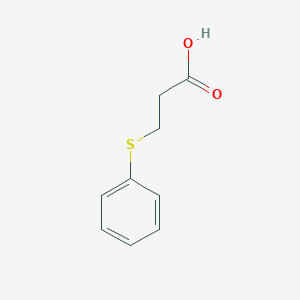
3-(Phenylthio)propanoic acid
Overview
Description
3-(Phenylthio)propanoic acid: is an organic compound with the molecular formula C9H10O2S . It is characterized by the presence of a phenylthio group attached to a propanoic acid backbone. This compound is known for its applications in various fields, including organic synthesis and medicinal chemistry.
Preparation Methods
Synthetic Routes and Reaction Conditions: The synthesis of 3-(Phenylthio)propanoic acid typically involves the reaction of thiophenol with acrylonitrile, followed by hydrolysis. The reaction conditions often include the use of a base such as sodium hydroxide or potassium hydroxide to facilitate the nucleophilic addition of thiophenol to acrylonitrile. The intermediate product is then hydrolyzed under acidic conditions to yield this compound.
Industrial Production Methods: In an industrial setting, the production of this compound may involve similar synthetic routes but on a larger scale. The process may be optimized for higher yields and purity, often involving continuous flow reactors and advanced purification techniques such as recrystallization or chromatography.
Chemical Reactions Analysis
Types of Reactions: 3-(Phenylthio)propanoic acid undergoes various chemical reactions, including:
Oxidation: The phenylthio group can be oxidized to form sulfoxides or sulfones using oxidizing agents such as hydrogen peroxide or m-chloroperbenzoic acid.
Reduction: The carboxylic acid group can be reduced to an alcohol using reducing agents like lithium aluminum hydride.
Substitution: The phenylthio group can participate in nucleophilic substitution reactions, where it can be replaced by other nucleophiles.
Common Reagents and Conditions:
Oxidation: Hydrogen peroxide, m-chloroperbenzoic acid, and other peroxides.
Reduction: Lithium aluminum hydride, sodium borohydride.
Substitution: Various nucleophiles such as amines, alcohols, and thiols.
Major Products:
Oxidation: Sulfoxides and sulfones.
Reduction: Alcohols.
Substitution: Substituted propanoic acids with different functional groups.
Scientific Research Applications
Chemistry: 3-(Phenylthio)propanoic acid is used as an intermediate in the synthesis of various organic compounds. It serves as a building block for the preparation of more complex molecules in organic synthesis.
Biology: In biological research, this compound is used to study enzyme interactions and metabolic pathways involving sulfur-containing compounds.
Medicine: This compound has potential applications in medicinal chemistry, particularly in the development of drugs that target specific enzymes or receptors. Its derivatives may exhibit pharmacological activities such as anti-inflammatory or antimicrobial properties.
Industry: In the industrial sector, this compound is used in the production of specialty chemicals and materials. It may also be employed in the synthesis of agrochemicals and pharmaceuticals.
Mechanism of Action
The mechanism of action of 3-(Phenylthio)propanoic acid involves its interaction with specific molecular targets, such as enzymes or receptors. The phenylthio group can participate in various biochemical reactions, influencing the activity of enzymes involved in metabolic pathways. The carboxylic acid group may also play a role in binding to target proteins, modulating their function.
Comparison with Similar Compounds
3-Phenylpropanoic acid: Lacks the sulfur atom, resulting in different chemical properties and reactivity.
3-(Phenylsulfanyl)propanoic acid: Similar structure but with a different sulfur oxidation state.
3-(Phenylthio)acetic acid: Similar functional groups but with a shorter carbon chain.
Uniqueness: 3-(Phenylthio)propanoic acid is unique due to the presence of both a phenylthio group and a propanoic acid backbone. This combination imparts distinct chemical reactivity and biological activity, making it valuable in various research and industrial applications.
Properties
IUPAC Name |
3-phenylsulfanylpropanoic acid | |
---|---|---|
Source | PubChem | |
URL | https://pubchem.ncbi.nlm.nih.gov | |
Description | Data deposited in or computed by PubChem | |
InChI |
InChI=1S/C9H10O2S/c10-9(11)6-7-12-8-4-2-1-3-5-8/h1-5H,6-7H2,(H,10,11) | |
Source | PubChem | |
URL | https://pubchem.ncbi.nlm.nih.gov | |
Description | Data deposited in or computed by PubChem | |
InChI Key |
IGPROYLOGZTOAM-UHFFFAOYSA-N | |
Source | PubChem | |
URL | https://pubchem.ncbi.nlm.nih.gov | |
Description | Data deposited in or computed by PubChem | |
Canonical SMILES |
C1=CC=C(C=C1)SCCC(=O)O | |
Source | PubChem | |
URL | https://pubchem.ncbi.nlm.nih.gov | |
Description | Data deposited in or computed by PubChem | |
Molecular Formula |
C9H10O2S | |
Source | PubChem | |
URL | https://pubchem.ncbi.nlm.nih.gov | |
Description | Data deposited in or computed by PubChem | |
DSSTOX Substance ID |
DTXSID80278629 | |
Record name | 3-(phenylthio)propanoic acid | |
Source | EPA DSSTox | |
URL | https://comptox.epa.gov/dashboard/DTXSID80278629 | |
Description | DSSTox provides a high quality public chemistry resource for supporting improved predictive toxicology. | |
Molecular Weight |
182.24 g/mol | |
Source | PubChem | |
URL | https://pubchem.ncbi.nlm.nih.gov | |
Description | Data deposited in or computed by PubChem | |
CAS No. |
5219-65-8 | |
Record name | 3-(Phenylthio)propanoic acid | |
Source | CAS Common Chemistry | |
URL | https://commonchemistry.cas.org/detail?cas_rn=5219-65-8 | |
Description | CAS Common Chemistry is an open community resource for accessing chemical information. Nearly 500,000 chemical substances from CAS REGISTRY cover areas of community interest, including common and frequently regulated chemicals, and those relevant to high school and undergraduate chemistry classes. This chemical information, curated by our expert scientists, is provided in alignment with our mission as a division of the American Chemical Society. | |
Explanation | The data from CAS Common Chemistry is provided under a CC-BY-NC 4.0 license, unless otherwise stated. | |
Record name | NSC 14777 | |
Source | ChemIDplus | |
URL | https://pubchem.ncbi.nlm.nih.gov/substance/?source=chemidplus&sourceid=0005219658 | |
Description | ChemIDplus is a free, web search system that provides access to the structure and nomenclature authority files used for the identification of chemical substances cited in National Library of Medicine (NLM) databases, including the TOXNET system. | |
Record name | 5219-65-8 | |
Source | DTP/NCI | |
URL | https://dtp.cancer.gov/dtpstandard/servlet/dwindex?searchtype=NSC&outputformat=html&searchlist=14777 | |
Description | The NCI Development Therapeutics Program (DTP) provides services and resources to the academic and private-sector research communities worldwide to facilitate the discovery and development of new cancer therapeutic agents. | |
Explanation | Unless otherwise indicated, all text within NCI products is free of copyright and may be reused without our permission. Credit the National Cancer Institute as the source. | |
Record name | 5219-65-8 | |
Source | DTP/NCI | |
URL | https://dtp.cancer.gov/dtpstandard/servlet/dwindex?searchtype=NSC&outputformat=html&searchlist=8542 | |
Description | The NCI Development Therapeutics Program (DTP) provides services and resources to the academic and private-sector research communities worldwide to facilitate the discovery and development of new cancer therapeutic agents. | |
Explanation | Unless otherwise indicated, all text within NCI products is free of copyright and may be reused without our permission. Credit the National Cancer Institute as the source. | |
Record name | 3-(phenylthio)propanoic acid | |
Source | EPA DSSTox | |
URL | https://comptox.epa.gov/dashboard/DTXSID80278629 | |
Description | DSSTox provides a high quality public chemistry resource for supporting improved predictive toxicology. | |
Record name | 3-(phenylsulfanyl)propanoic acid | |
Source | European Chemicals Agency (ECHA) | |
URL | https://echa.europa.eu/information-on-chemicals | |
Description | The European Chemicals Agency (ECHA) is an agency of the European Union which is the driving force among regulatory authorities in implementing the EU's groundbreaking chemicals legislation for the benefit of human health and the environment as well as for innovation and competitiveness. | |
Explanation | Use of the information, documents and data from the ECHA website is subject to the terms and conditions of this Legal Notice, and subject to other binding limitations provided for under applicable law, the information, documents and data made available on the ECHA website may be reproduced, distributed and/or used, totally or in part, for non-commercial purposes provided that ECHA is acknowledged as the source: "Source: European Chemicals Agency, http://echa.europa.eu/". Such acknowledgement must be included in each copy of the material. ECHA permits and encourages organisations and individuals to create links to the ECHA website under the following cumulative conditions: Links can only be made to webpages that provide a link to the Legal Notice page. | |
Synthesis routes and methods
Procedure details
Retrosynthesis Analysis
AI-Powered Synthesis Planning: Our tool employs the Template_relevance Pistachio, Template_relevance Bkms_metabolic, Template_relevance Pistachio_ringbreaker, Template_relevance Reaxys, Template_relevance Reaxys_biocatalysis model, leveraging a vast database of chemical reactions to predict feasible synthetic routes.
One-Step Synthesis Focus: Specifically designed for one-step synthesis, it provides concise and direct routes for your target compounds, streamlining the synthesis process.
Accurate Predictions: Utilizing the extensive PISTACHIO, BKMS_METABOLIC, PISTACHIO_RINGBREAKER, REAXYS, REAXYS_BIOCATALYSIS database, our tool offers high-accuracy predictions, reflecting the latest in chemical research and data.
Strategy Settings
Precursor scoring | Relevance Heuristic |
---|---|
Min. plausibility | 0.01 |
Model | Template_relevance |
Template Set | Pistachio/Bkms_metabolic/Pistachio_ringbreaker/Reaxys/Reaxys_biocatalysis |
Top-N result to add to graph | 6 |
Feasible Synthetic Routes
Disclaimer and Information on In-Vitro Research Products
Please be aware that all articles and product information presented on BenchChem are intended solely for informational purposes. The products available for purchase on BenchChem are specifically designed for in-vitro studies, which are conducted outside of living organisms. In-vitro studies, derived from the Latin term "in glass," involve experiments performed in controlled laboratory settings using cells or tissues. It is important to note that these products are not categorized as medicines or drugs, and they have not received approval from the FDA for the prevention, treatment, or cure of any medical condition, ailment, or disease. We must emphasize that any form of bodily introduction of these products into humans or animals is strictly prohibited by law. It is essential to adhere to these guidelines to ensure compliance with legal and ethical standards in research and experimentation.