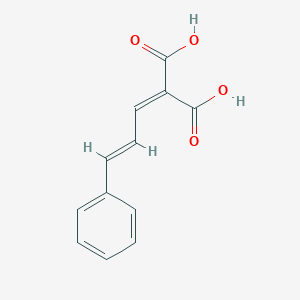
Cinnamylidenemalonic acid
Overview
Description
Cinnamylidenemalonic acid is an organic compound with the chemical formula C12H10O4. It is characterized by a white or light yellow crystalline powder appearance and has a melting point of 132-134 degrees Celsius . This compound is known for its applications in organic synthesis reactions and its role in the synthesis of various drugs and organic compounds .
Mechanism of Action
Target of Action
Cinnamylidenemalonic acid has been found to exhibit antimicrobial activity, with its primary targets being pathogenic fungi and bacteria . In Candida albicans, the most likely targets of the compound are caHOS2 and caRPD3 . In Staphylococcus aureus, the most likely target is saFABH .
Mode of Action
The compound interacts directly with the ergosterol present in the fungal plasmatic membrane and with the cell wall . This interaction disrupts the integrity of the cell wall and membrane, leading to the death of the fungal cells .
Biochemical Pathways
This compound is involved in the decarboxylation process . The compound undergoes decarboxylation in resorcinol and catechol, a process that has been studied kinetically . The activation parameters for this process have been calculated and compared with those previously reported for the decarboxylation of unsubstituted and substituted malonic acids .
Pharmacokinetics
The compound’s antimicrobial activity suggests that it is able to reach its targets in sufficient concentrations to exert its effects .
Result of Action
The result of this compound’s action is the death of pathogenic fungi and bacteria . The compound’s interaction with the cell wall and membrane of these organisms disrupts their integrity, leading to cell death .
Action Environment
This compound was found to crystallize in three different polymorphic structures, each of which underwent a photochemical reaction when irradiated with 257.3A of light . This suggests that the compound’s action, efficacy, and stability may be influenced by environmental factors such as light exposure .
Biochemical Analysis
Biochemical Properties
Cinnamylidenemalonic acid plays a significant role in biochemical reactions, particularly in organic synthesis. It can participate in ketonization reactions of halogenated hydrocarbons and acetylation reactions of aromatic aldehydes . The compound interacts with various enzymes and proteins during these reactions, although specific enzymes and proteins involved are not well-documented in the literature. The nature of these interactions typically involves the formation of intermediate compounds that facilitate the desired chemical transformations.
Cellular Effects
The effects of this compound on various types of cells and cellular processes are not extensively studied. It is known that cinnamic acid derivatives, which are structurally related to this compound, can exhibit anticancer properties by inducing cell death in colon and cervical cancer cells . These effects are likely mediated through the inhibition of histone deacetylases (HDACs), leading to changes in gene expression and cellular metabolism.
Molecular Mechanism
The molecular mechanism of this compound involves its participation in photochemical reactions and dimerization processes. When exposed to light, this compound can undergo [2+2] dimerization, forming cyclobutane derivatives . This reaction is facilitated by the compound’s ability to absorb light and form reactive intermediates that can interact with other molecules. Additionally, this compound can undergo decarboxylation in the presence of resorcinol and catechol, leading to the formation of conjugated diene-acids .
Temporal Effects in Laboratory Settings
In laboratory settings, the stability and degradation of this compound have been studied. The compound is relatively stable at room temperature and does not easily explode or burn . It can undergo decarboxylation when heated, leading to the formation of different products over time . Long-term effects on cellular function have not been extensively documented, but the compound’s stability suggests that it can be used in various experimental conditions without significant degradation.
Dosage Effects in Animal Models
The effects of this compound at different dosages in animal models have not been well-documented. Studies on related compounds suggest that dosage can significantly impact the compound’s efficacy and toxicity. For example, high doses of cinnamic acid derivatives can exhibit toxic effects, while lower doses may have beneficial properties
Metabolic Pathways
This compound is involved in various metabolic pathways, particularly those related to organic synthesis. The compound can be synthesized from cinnamic acid and dichloromethane under alkaline conditions . It may also participate in metabolic pathways involving short-chain fatty acids, which play a crucial role in regulating glucose and lipid metabolism
Transport and Distribution
The transport and distribution of this compound within cells and tissues are not extensively studied. It is likely that the compound can be transported across cell membranes through passive diffusion or facilitated transport mechanisms. Binding proteins and transporters specific to this compound have not been identified, but the compound’s solubility in organic solvents suggests that it can be distributed within lipid-rich environments .
Subcellular Localization
The subcellular localization of this compound is not well-documented. Given its solubility properties, it is likely to localize within lipid-rich compartments such as the endoplasmic reticulum and lipid droplets. The compound may also interact with specific targeting signals or undergo post-translational modifications that direct it to particular subcellular compartments .
Preparation Methods
Synthetic Routes and Reaction Conditions: Cinnamylidenemalonic acid is typically synthesized by reacting cinnamic acid with dichloromethane under alkaline conditions. The reaction involves the use of sodium hydroxide as a base, resulting in the formation of this compound along with sodium chloride and water as by-products .
Industrial Production Methods: While specific industrial production methods are not extensively documented, the preparation method mentioned above can be scaled up for industrial purposes. The reaction conditions, such as temperature and concentration of reactants, can be optimized to achieve higher yields and purity of the final product.
Chemical Reactions Analysis
Types of Reactions: Cinnamylidenemalonic acid undergoes various chemical reactions, including:
Decarboxylation: When heated in the presence of quinoline at 130°C, this compound undergoes decarboxylation to form 5-phenylpenta-2(Z),4(E)-dienoic acid.
Photochemical Reactions: The compound can undergo photochemical reactions when irradiated with specific wavelengths of light, leading to the formation of different products depending on the polymorphic structure of the compound.
Common Reagents and Conditions:
Decarboxylation: Quinoline is commonly used as a catalyst for the decarboxylation reaction.
Photochemical Reactions: Specific wavelengths of light are used to induce photochemical reactions.
Major Products Formed:
Decarboxylation: 5-phenylpenta-2(Z),4(E)-dienoic acid.
Photochemical Reactions: Various products depending on the polymorphic structure and reaction conditions.
Scientific Research Applications
Cinnamylidenemalonic acid has several scientific research applications, including:
Organic Synthesis: It is used in organic synthesis reactions, such as the ketonization reaction of halogenated hydrocarbons and the acetylation reaction of aromatic aldehydes.
Drug Synthesis: The compound is utilized in the synthesis of drugs and organic compounds, including cinnamic acid and cinnamaldehyde.
Photochemical Studies:
Comparison with Similar Compounds
- Malonic Acid
- Cinnamic Acid
- Ethylidenemalonic Acid
Properties
IUPAC Name |
2-cinnamylidenepropanedioic acid | |
---|---|---|
Details | Computed by Lexichem TK 2.7.0 (PubChem release 2021.05.07) | |
Source | PubChem | |
URL | https://pubchem.ncbi.nlm.nih.gov | |
Description | Data deposited in or computed by PubChem | |
InChI |
InChI=1S/C12H10O4/c13-11(14)10(12(15)16)8-4-7-9-5-2-1-3-6-9/h1-8H,(H,13,14)(H,15,16) | |
Details | Computed by InChI 1.0.6 (PubChem release 2021.05.07) | |
Source | PubChem | |
URL | https://pubchem.ncbi.nlm.nih.gov | |
Description | Data deposited in or computed by PubChem | |
InChI Key |
BLPUXJIIRIWMSQ-UHFFFAOYSA-N | |
Details | Computed by InChI 1.0.6 (PubChem release 2021.05.07) | |
Source | PubChem | |
URL | https://pubchem.ncbi.nlm.nih.gov | |
Description | Data deposited in or computed by PubChem | |
Canonical SMILES |
C1=CC=C(C=C1)C=CC=C(C(=O)O)C(=O)O | |
Details | Computed by OEChem 2.3.0 (PubChem release 2021.05.07) | |
Source | PubChem | |
URL | https://pubchem.ncbi.nlm.nih.gov | |
Description | Data deposited in or computed by PubChem | |
Molecular Formula |
C12H10O4 | |
Details | Computed by PubChem 2.1 (PubChem release 2021.05.07) | |
Source | PubChem | |
URL | https://pubchem.ncbi.nlm.nih.gov | |
Description | Data deposited in or computed by PubChem | |
DSSTOX Substance ID |
DTXSID201240229 | |
Record name | 2-(3-Phenyl-2-propen-1-ylidene)propanedioic acid | |
Source | EPA DSSTox | |
URL | https://comptox.epa.gov/dashboard/DTXSID201240229 | |
Description | DSSTox provides a high quality public chemistry resource for supporting improved predictive toxicology. | |
Molecular Weight |
218.20 g/mol | |
Details | Computed by PubChem 2.1 (PubChem release 2021.05.07) | |
Source | PubChem | |
URL | https://pubchem.ncbi.nlm.nih.gov | |
Description | Data deposited in or computed by PubChem | |
CAS No. |
4472-92-8 | |
Record name | 2-(3-Phenyl-2-propen-1-ylidene)propanedioic acid | |
Source | CAS Common Chemistry | |
URL | https://commonchemistry.cas.org/detail?cas_rn=4472-92-8 | |
Description | CAS Common Chemistry is an open community resource for accessing chemical information. Nearly 500,000 chemical substances from CAS REGISTRY cover areas of community interest, including common and frequently regulated chemicals, and those relevant to high school and undergraduate chemistry classes. This chemical information, curated by our expert scientists, is provided in alignment with our mission as a division of the American Chemical Society. | |
Explanation | The data from CAS Common Chemistry is provided under a CC-BY-NC 4.0 license, unless otherwise stated. | |
Record name | 2-(3-Phenyl-2-propen-1-ylidene)propanedioic acid | |
Source | EPA DSSTox | |
URL | https://comptox.epa.gov/dashboard/DTXSID201240229 | |
Description | DSSTox provides a high quality public chemistry resource for supporting improved predictive toxicology. | |
Retrosynthesis Analysis
AI-Powered Synthesis Planning: Our tool employs the Template_relevance Pistachio, Template_relevance Bkms_metabolic, Template_relevance Pistachio_ringbreaker, Template_relevance Reaxys, Template_relevance Reaxys_biocatalysis model, leveraging a vast database of chemical reactions to predict feasible synthetic routes.
One-Step Synthesis Focus: Specifically designed for one-step synthesis, it provides concise and direct routes for your target compounds, streamlining the synthesis process.
Accurate Predictions: Utilizing the extensive PISTACHIO, BKMS_METABOLIC, PISTACHIO_RINGBREAKER, REAXYS, REAXYS_BIOCATALYSIS database, our tool offers high-accuracy predictions, reflecting the latest in chemical research and data.
Strategy Settings
Precursor scoring | Relevance Heuristic |
---|---|
Min. plausibility | 0.01 |
Model | Template_relevance |
Template Set | Pistachio/Bkms_metabolic/Pistachio_ringbreaker/Reaxys/Reaxys_biocatalysis |
Top-N result to add to graph | 6 |
Feasible Synthetic Routes
Q1: What are the different polymorphic structures of cinnamylidenemalonic acid and how do they react to UV irradiation?
A: this compound has been found to crystallize in three distinct polymorphic forms. When exposed to UV light at 257.3 Å, each polymorph exhibits unique photochemical reactivity []. Polymorph 1 yields a mixture of products upon irradiation. In contrast, both polymorphs 2 and 3 undergo a [2+2] dimerization reaction, resulting in the formation of the same dimer product [].
Q2: Can you elaborate on the mechanism of decarboxylation of this compound in the presence of pyridine?
A: Research suggests that the decarboxylation of this compound in pyridine involves a dual pathway mechanism []. This conclusion stems from experiments using carboxy-labelled dideuteriomalonic acid. Both the resulting 5-phenylpenta-2(Z),4(E)-dienoic acid and 5-phenylpenta-2(E),4(E)-dienoic acid isomers exhibit a similar ~2:1 α/γ deuterium labelling pattern, suggesting a common deuteriated lactone intermediate in both pathways [].
Disclaimer and Information on In-Vitro Research Products
Please be aware that all articles and product information presented on BenchChem are intended solely for informational purposes. The products available for purchase on BenchChem are specifically designed for in-vitro studies, which are conducted outside of living organisms. In-vitro studies, derived from the Latin term "in glass," involve experiments performed in controlled laboratory settings using cells or tissues. It is important to note that these products are not categorized as medicines or drugs, and they have not received approval from the FDA for the prevention, treatment, or cure of any medical condition, ailment, or disease. We must emphasize that any form of bodily introduction of these products into humans or animals is strictly prohibited by law. It is essential to adhere to these guidelines to ensure compliance with legal and ethical standards in research and experimentation.