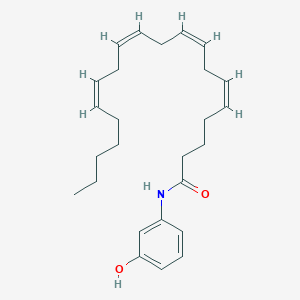
N-(3-hydroxyphenyl)-Arachidonoyl amide
Overview
Description
N-(3-hydroxyphenyl)-Arachidonoyl amide: is a chemical compound that belongs to the class of amides. It is derived from arachidonic acid and 3-hydroxyaniline. This compound is known for its biological activities and has been studied for its potential therapeutic applications.
Mechanism of Action
Target of Action
N-(3-hydroxyphenyl)-Arachidonoyl amide, also known as AM404, is a potent agonist of the transient receptor potential vanilloid type 1 (TRPV1) and a low-affinity ligand of the cannabinoid receptor type 1 (CB1) . These receptors play crucial roles in pain perception, inflammation, and body temperature regulation .
Mode of Action
AM404 interacts with its targets, TRPV1 and CB1 receptors, leading to a series of intracellular events. The interaction with TRPV1 receptors can lead to the influx of calcium ions, which can further trigger various cellular responses. The binding to CB1 receptors, on the other hand, can inhibit adenylate cyclase activity, reducing the levels of cyclic AMP and modulating the activity of protein kinase A .
Biochemical Pathways
AM404 affects the arachidonic acid pathway, a key biochemical pathway involved in inflammation and pain sensation. It inhibits the activity of cyclooxygenase-1 (COX-1) and cyclooxygenase-2 (COX-2), enzymes that catalyze the conversion of arachidonic acid to prostaglandins . This inhibition leads to a decrease in the production of prostaglandins, bioactive lipids that play a key role in inflammation and pain .
Pharmacokinetics
In the CNS, p-aminophenol is conjugated with arachidonic acid by the fatty acid amide hydrolase (FAAH) to produce AM404 .
Result of Action
The primary result of AM404’s action is the reduction of inflammation and pain sensation. By inhibiting the production of prostaglandins, AM404 can decrease inflammation and pain. Moreover, it also inhibits the formation of reactive oxygen species, further contributing to its anti-inflammatory effects .
Action Environment
The action of AM404 can be influenced by various environmental factors. For instance, the presence of other inflammatory mediators can modulate the activity of AM404. Additionally, the pH of the environment can affect the ionization state of AM404, potentially influencing its interaction with its targets .
Biochemical Analysis
Biochemical Properties
N-(3-hydroxyphenyl)-Arachidonoyl amide interacts with several enzymes, proteins, and other biomolecules. It is known to exert its pharmacological effects in immune cells
Cellular Effects
The cellular effects of this compound are diverse and significant. It influences cell function, including impacts on cell signaling pathways, gene expression, and cellular metabolism . For instance, it has been shown to prevent prostaglandin synthesis in activated microglia by inhibiting COX activity .
Molecular Mechanism
The molecular mechanism of this compound involves binding interactions with biomolecules, enzyme inhibition or activation, and changes in gene expression . It is a potent agonist of the TRPV1 and a low-affinity ligand of the CB1 .
Metabolic Pathways
This compound is involved in several metabolic pathways. It interacts with enzymes or cofactors and can affect metabolic flux or metabolite levels
Preparation Methods
Synthetic Routes and Reaction Conditions: The synthesis of N-(3-hydroxyphenyl)-Arachidonoyl amide typically involves the reaction of arachidonic acid with 3-hydroxyaniline. The reaction is usually carried out in the presence of a coupling agent such as N,N’-dicyclohexylcarbodiimide (DCC) and a catalyst like 4-dimethylaminopyridine (DMAP). The reaction is conducted under an inert atmosphere, typically nitrogen, and at a temperature range of 0-25°C. The product is then purified using column chromatography.
Industrial Production Methods: Industrial production of this compound follows similar synthetic routes but on a larger scale. The process involves the use of large reactors and automated systems to control the reaction conditions precisely. The purification process may involve crystallization or large-scale chromatography to ensure high purity of the final product.
Chemical Reactions Analysis
Types of Reactions:
Oxidation: N-(3-hydroxyphenyl)-Arachidonoyl amide can undergo oxidation reactions, leading to the formation of quinone derivatives.
Reduction: The compound can be reduced to form corresponding amines.
Substitution: It can participate in substitution reactions, particularly nucleophilic substitutions, where the hydroxyl group can be replaced by other nucleophiles.
Common Reagents and Conditions:
Oxidation: Common oxidizing agents include potassium permanganate (KMnO₄) and chromium trioxide (CrO₃).
Reduction: Reducing agents such as lithium aluminum hydride (LiAlH₄) or sodium borohydride (NaBH₄) are used.
Substitution: Nucleophiles like halides, thiols, or amines can be used under basic or acidic conditions.
Major Products:
Oxidation: Quinone derivatives.
Reduction: Corresponding amines.
Substitution: Various substituted derivatives depending on the nucleophile used.
Scientific Research Applications
Chemistry: N-(3-hydroxyphenyl)-Arachidonoyl amide is used as a precursor in the synthesis of various bioactive molecules. It serves as an intermediate in organic synthesis and is used in the development of new chemical entities.
Biology: In biological research, this compound is studied for its role in cellular signaling pathways. It is known to interact with various receptors and enzymes, influencing cellular processes.
Medicine: this compound has potential therapeutic applications. It is investigated for its anti-inflammatory, analgesic, and neuroprotective properties. It is also studied for its potential use in treating conditions like chronic pain and neurodegenerative diseases.
Industry: In the industrial sector, this compound is used in the formulation of pharmaceuticals and as a research chemical in the development of new drugs.
Comparison with Similar Compounds
N-arachidonoyl dopamine (NADA): Similar in structure but contains a dopamine moiety instead of a hydroxyphenyl group.
N-arachidonoyl serotonin (NAS): Contains a serotonin moiety.
N-arachidonoyl glycine (NAGly): Contains a glycine moiety.
Uniqueness: N-(3-hydroxyphenyl)-Arachidonoyl amide is unique due to its specific interaction with TRPV1 and CB1 receptors, which distinguishes it from other similar compounds. Its hydroxyl group also provides additional sites for chemical modifications, enhancing its versatility in research and therapeutic applications.
Properties
IUPAC Name |
(5Z,8Z,11Z,14Z)-N-(3-hydroxyphenyl)icosa-5,8,11,14-tetraenamide | |
---|---|---|
Source | PubChem | |
URL | https://pubchem.ncbi.nlm.nih.gov | |
Description | Data deposited in or computed by PubChem | |
InChI |
InChI=1S/C26H37NO2/c1-2-3-4-5-6-7-8-9-10-11-12-13-14-15-16-17-18-22-26(29)27-24-20-19-21-25(28)23-24/h6-7,9-10,12-13,15-16,19-21,23,28H,2-5,8,11,14,17-18,22H2,1H3,(H,27,29)/b7-6-,10-9-,13-12-,16-15- | |
Source | PubChem | |
URL | https://pubchem.ncbi.nlm.nih.gov | |
Description | Data deposited in or computed by PubChem | |
InChI Key |
ZWSMMQKRRVRXBA-DOFZRALJSA-N | |
Source | PubChem | |
URL | https://pubchem.ncbi.nlm.nih.gov | |
Description | Data deposited in or computed by PubChem | |
Canonical SMILES |
CCCCCC=CCC=CCC=CCC=CCCCC(=O)NC1=CC(=CC=C1)O | |
Source | PubChem | |
URL | https://pubchem.ncbi.nlm.nih.gov | |
Description | Data deposited in or computed by PubChem | |
Isomeric SMILES |
CCCCC/C=C\C/C=C\C/C=C\C/C=C\CCCC(=O)NC1=CC(=CC=C1)O | |
Source | PubChem | |
URL | https://pubchem.ncbi.nlm.nih.gov | |
Description | Data deposited in or computed by PubChem | |
Molecular Formula |
C26H37NO2 | |
Source | PubChem | |
URL | https://pubchem.ncbi.nlm.nih.gov | |
Description | Data deposited in or computed by PubChem | |
Molecular Weight |
395.6 g/mol | |
Source | PubChem | |
URL | https://pubchem.ncbi.nlm.nih.gov | |
Description | Data deposited in or computed by PubChem | |
Retrosynthesis Analysis
AI-Powered Synthesis Planning: Our tool employs the Template_relevance Pistachio, Template_relevance Bkms_metabolic, Template_relevance Pistachio_ringbreaker, Template_relevance Reaxys, Template_relevance Reaxys_biocatalysis model, leveraging a vast database of chemical reactions to predict feasible synthetic routes.
One-Step Synthesis Focus: Specifically designed for one-step synthesis, it provides concise and direct routes for your target compounds, streamlining the synthesis process.
Accurate Predictions: Utilizing the extensive PISTACHIO, BKMS_METABOLIC, PISTACHIO_RINGBREAKER, REAXYS, REAXYS_BIOCATALYSIS database, our tool offers high-accuracy predictions, reflecting the latest in chemical research and data.
Strategy Settings
Precursor scoring | Relevance Heuristic |
---|---|
Min. plausibility | 0.01 |
Model | Template_relevance |
Template Set | Pistachio/Bkms_metabolic/Pistachio_ringbreaker/Reaxys/Reaxys_biocatalysis |
Top-N result to add to graph | 6 |
Feasible Synthetic Routes
Disclaimer and Information on In-Vitro Research Products
Please be aware that all articles and product information presented on BenchChem are intended solely for informational purposes. The products available for purchase on BenchChem are specifically designed for in-vitro studies, which are conducted outside of living organisms. In-vitro studies, derived from the Latin term "in glass," involve experiments performed in controlled laboratory settings using cells or tissues. It is important to note that these products are not categorized as medicines or drugs, and they have not received approval from the FDA for the prevention, treatment, or cure of any medical condition, ailment, or disease. We must emphasize that any form of bodily introduction of these products into humans or animals is strictly prohibited by law. It is essential to adhere to these guidelines to ensure compliance with legal and ethical standards in research and experimentation.