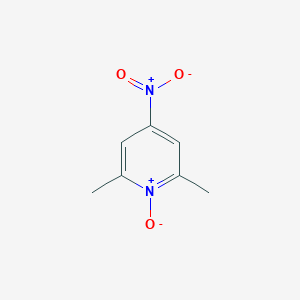
2,6-Dimethyl-4-nitropyridine 1-oxide
Overview
Description
2,6-Dimethyl-4-nitropyridine 1-oxide is a chemical compound with the molecular formula C₇H₈N₂O₃ It is a derivative of pyridine, characterized by the presence of two methyl groups at positions 2 and 6, a nitro group at position 4, and an N-oxide functional group
Mechanism of Action
Target of Action
It’s known that nitropyridine-1-oxides, in general, are excellent π-hole donors . They participate in several interactions like hydrogen-bonding (HB), halogen-bonding (XB), triel bonding (TrB), and coordination-bonding (CB) (N+–O− coordinated to a transition metal) .
Mode of Action
The compound interacts with its targets primarily through π-hole interactions via the nitro group . Simultaneously, the N-oxide group participates in a series of interactions as an electron donor . The molecular electrostatic potential (MEP) value above and below the π–hole of the nitro group is largely influenced by the participation of the N-oxide group in these interactions .
Biochemical Pathways
It’s known that the pathway to 4-nitropyridine via the pyridine n-oxide involves two steps, including a nitration step and a reduction step .
Result of Action
It’s known that the compound forms a self-assembled tetramer through a double π-hole interaction established between the nitro group .
Action Environment
Environmental factors can influence the action, efficacy, and stability of 2,6-Dimethyl-4-nitropyridine 1-oxide. For instance, the compound is a potentially explosive nitration product . Therefore, the continuous flow methodology is used to minimize accumulation and ensure safe scale-up . Additionally, the compound is a combustible substance and can explode in air . It is almost insoluble in water but can dissolve in organic solvents .
Preparation Methods
Synthetic Routes and Reaction Conditions: The synthesis of 2,6-Dimethyl-4-nitropyridine 1-oxide typically involves the nitration of 2,6-dimethylpyridine N-oxide. One common method includes dissolving 2,6-dimethylpyridine N-oxide in concentrated sulfuric acid, followed by the addition of a nitrating agent such as nitric acid. The reaction is carried out under controlled temperature conditions to ensure the selective formation of the nitro compound .
Industrial Production Methods: In industrial settings, the production of this compound may utilize continuous flow synthesis techniques. This approach minimizes the risks associated with the handling of highly energetic nitration products and allows for efficient scale-up. The continuous flow methodology ensures better control over reaction conditions, leading to higher yields and selectivity .
Chemical Reactions Analysis
Types of Reactions: 2,6-Dimethyl-4-nitropyridine 1-oxide undergoes various chemical reactions, including:
Oxidation: The N-oxide group can participate in oxidation reactions, often facilitated by oxidizing agents such as hydrogen peroxide.
Reduction: The nitro group can be reduced to an amino group using reducing agents like hydrogen gas in the presence of a catalyst.
Substitution: The compound can undergo nucleophilic substitution reactions, where the nitro group is replaced by other functional groups.
Common Reagents and Conditions:
Oxidation: Hydrogen peroxide, acetic acid.
Reduction: Hydrogen gas, palladium on carbon (Pd/C) catalyst.
Substitution: Nucleophiles such as amines or thiols.
Major Products:
Oxidation: Formation of higher oxidation state compounds.
Reduction: Formation of 2,6-dimethyl-4-aminopyridine 1-oxide.
Substitution: Formation of various substituted pyridine derivatives.
Scientific Research Applications
2,6-Dimethyl-4-nitropyridine 1-oxide has several applications in scientific research:
Chemistry: Used as a building block for the synthesis of more complex organic molecules.
Biology: Investigated for its potential biological activities and interactions with biomolecules.
Medicine: Explored for its potential use in drug development and as a pharmacophore in medicinal chemistry.
Industry: Utilized in the production of specialty chemicals and materials.
Comparison with Similar Compounds
- 2,3-Dimethyl-4-nitropyridine 1-oxide
- 3,5-Dimethyl-4-nitropyridine 1-oxide
- 4-Nitropyridine N-oxide
Comparison: 2,6-Dimethyl-4-nitropyridine 1-oxide is unique due to the specific positioning of its methyl and nitro groups, which influence its chemical reactivity and interactions.
Properties
IUPAC Name |
2,6-dimethyl-4-nitro-1-oxidopyridin-1-ium | |
---|---|---|
Source | PubChem | |
URL | https://pubchem.ncbi.nlm.nih.gov | |
Description | Data deposited in or computed by PubChem | |
InChI |
InChI=1S/C7H8N2O3/c1-5-3-7(9(11)12)4-6(2)8(5)10/h3-4H,1-2H3 | |
Source | PubChem | |
URL | https://pubchem.ncbi.nlm.nih.gov | |
Description | Data deposited in or computed by PubChem | |
InChI Key |
LFPUGENPUAERSG-UHFFFAOYSA-N | |
Source | PubChem | |
URL | https://pubchem.ncbi.nlm.nih.gov | |
Description | Data deposited in or computed by PubChem | |
Canonical SMILES |
CC1=CC(=CC(=[N+]1[O-])C)[N+](=O)[O-] | |
Source | PubChem | |
URL | https://pubchem.ncbi.nlm.nih.gov | |
Description | Data deposited in or computed by PubChem | |
Molecular Formula |
C7H8N2O3 | |
Source | PubChem | |
URL | https://pubchem.ncbi.nlm.nih.gov | |
Description | Data deposited in or computed by PubChem | |
DSSTOX Substance ID |
DTXSID70197411 | |
Record name | 2,6-Lutidine, 4-nitro-, 1-oxide | |
Source | EPA DSSTox | |
URL | https://comptox.epa.gov/dashboard/DTXSID70197411 | |
Description | DSSTox provides a high quality public chemistry resource for supporting improved predictive toxicology. | |
Molecular Weight |
168.15 g/mol | |
Source | PubChem | |
URL | https://pubchem.ncbi.nlm.nih.gov | |
Description | Data deposited in or computed by PubChem | |
CAS No. |
4808-64-4 | |
Record name | Pyridine, 2,6-dimethyl-4-nitro-, 1-oxide | |
Source | CAS Common Chemistry | |
URL | https://commonchemistry.cas.org/detail?cas_rn=4808-64-4 | |
Description | CAS Common Chemistry is an open community resource for accessing chemical information. Nearly 500,000 chemical substances from CAS REGISTRY cover areas of community interest, including common and frequently regulated chemicals, and those relevant to high school and undergraduate chemistry classes. This chemical information, curated by our expert scientists, is provided in alignment with our mission as a division of the American Chemical Society. | |
Explanation | The data from CAS Common Chemistry is provided under a CC-BY-NC 4.0 license, unless otherwise stated. | |
Record name | 4-Nitro-2,6-lutidine-1-oxide | |
Source | ChemIDplus | |
URL | https://pubchem.ncbi.nlm.nih.gov/substance/?source=chemidplus&sourceid=0004808644 | |
Description | ChemIDplus is a free, web search system that provides access to the structure and nomenclature authority files used for the identification of chemical substances cited in National Library of Medicine (NLM) databases, including the TOXNET system. | |
Record name | 4808-64-4 | |
Source | DTP/NCI | |
URL | https://dtp.cancer.gov/dtpstandard/servlet/dwindex?searchtype=NSC&outputformat=html&searchlist=5094 | |
Description | The NCI Development Therapeutics Program (DTP) provides services and resources to the academic and private-sector research communities worldwide to facilitate the discovery and development of new cancer therapeutic agents. | |
Explanation | Unless otherwise indicated, all text within NCI products is free of copyright and may be reused without our permission. Credit the National Cancer Institute as the source. | |
Record name | 2,6-Lutidine, 4-nitro-, 1-oxide | |
Source | EPA DSSTox | |
URL | https://comptox.epa.gov/dashboard/DTXSID70197411 | |
Description | DSSTox provides a high quality public chemistry resource for supporting improved predictive toxicology. | |
Record name | 4-Nitro-2,6-lutidine-1-oxide | |
Source | FDA Global Substance Registration System (GSRS) | |
URL | https://gsrs.ncats.nih.gov/ginas/app/beta/substances/25F79GKV74 | |
Description | The FDA Global Substance Registration System (GSRS) enables the efficient and accurate exchange of information on what substances are in regulated products. Instead of relying on names, which vary across regulatory domains, countries, and regions, the GSRS knowledge base makes it possible for substances to be defined by standardized, scientific descriptions. | |
Explanation | Unless otherwise noted, the contents of the FDA website (www.fda.gov), both text and graphics, are not copyrighted. They are in the public domain and may be republished, reprinted and otherwise used freely by anyone without the need to obtain permission from FDA. Credit to the U.S. Food and Drug Administration as the source is appreciated but not required. | |
Synthesis routes and methods I
Procedure details
Synthesis routes and methods II
Procedure details
Synthesis routes and methods III
Procedure details
Retrosynthesis Analysis
AI-Powered Synthesis Planning: Our tool employs the Template_relevance Pistachio, Template_relevance Bkms_metabolic, Template_relevance Pistachio_ringbreaker, Template_relevance Reaxys, Template_relevance Reaxys_biocatalysis model, leveraging a vast database of chemical reactions to predict feasible synthetic routes.
One-Step Synthesis Focus: Specifically designed for one-step synthesis, it provides concise and direct routes for your target compounds, streamlining the synthesis process.
Accurate Predictions: Utilizing the extensive PISTACHIO, BKMS_METABOLIC, PISTACHIO_RINGBREAKER, REAXYS, REAXYS_BIOCATALYSIS database, our tool offers high-accuracy predictions, reflecting the latest in chemical research and data.
Strategy Settings
Precursor scoring | Relevance Heuristic |
---|---|
Min. plausibility | 0.01 |
Model | Template_relevance |
Template Set | Pistachio/Bkms_metabolic/Pistachio_ringbreaker/Reaxys/Reaxys_biocatalysis |
Top-N result to add to graph | 6 |
Feasible Synthetic Routes
Q1: What makes the 4-position of 2,6-Dimethyl-4-nitropyridine N-oxide particularly reactive?
A: The nitro group at the 4-position, along with the N-oxide group, significantly enhances the reactivity of this position towards nucleophilic substitution reactions. This is due to the strong electron-withdrawing nature of both groups, which increases the electrophilicity of the 4-position and facilitates nucleophilic attack. []
Q2: Can you provide an example of how the nitro group in 2,6-Dimethyl-4-nitropyridine N-oxide is utilized in synthesis?
A: Research has shown that the nitro group in 2,6-Dimethyl-4-nitropyridine N-oxide can be easily replaced with a methylthio group using sodium methanethiolate. This reaction yields 3-halo-2,6-dimethyl-4-methylthiopyridine 1-oxides. The presence of a halogen atom adjacent to the nitro group further enhances the reactivity. []
Q3: How does the presence of the N-oxide moiety in 2,6-Dimethyl-4-nitropyridine N-oxide influence its crystal packing?
A: Studies have revealed that the N-oxide moiety plays a crucial role in the crystal structure of 2,6-Dimethyl-4-nitropyridine N-oxide. It participates in attractive intermolecular C–H⋯O interactions, influencing the overall packing arrangement within the crystal lattice. This interaction is particularly observed in the crystal structures of 3-chloro- and 3-bromo-2,6-dimethyl-4-nitropyridine N-oxide. [, ]
Q4: Have there been any computational studies conducted on 2,6-Dimethyl-4-nitropyridine N-oxide?
A: Yes, density functional theory (DFT) calculations have been employed to characterize 2,6-Dimethyl-4-nitropyridine N-oxide. [] These calculations provide valuable insights into the electronic structure, vibrational frequencies, and other molecular properties.
Q5: What synthetic strategies have been explored using 2,6-Dimethyl-4-nitropyridine N-oxide?
A: Researchers have investigated the Reissert-Kaufmann-type reaction using 2,6-Dimethyl-4-nitropyridine N-oxide as a starting material. [] This reaction is a valuable method for the synthesis of substituted heterocycles.
Q6: Is there information available on the vibrational properties of 2,6-Dimethyl-4-nitropyridine N-oxide derivatives?
A: Yes, the vibrational properties of 3-iodo-2,6-dimethyl-4-nitropyridine N-oxide have been studied, along with its X-ray crystal structure. [] This research provides insights into the molecular vibrations and structural features of this derivative.
Disclaimer and Information on In-Vitro Research Products
Please be aware that all articles and product information presented on BenchChem are intended solely for informational purposes. The products available for purchase on BenchChem are specifically designed for in-vitro studies, which are conducted outside of living organisms. In-vitro studies, derived from the Latin term "in glass," involve experiments performed in controlled laboratory settings using cells or tissues. It is important to note that these products are not categorized as medicines or drugs, and they have not received approval from the FDA for the prevention, treatment, or cure of any medical condition, ailment, or disease. We must emphasize that any form of bodily introduction of these products into humans or animals is strictly prohibited by law. It is essential to adhere to these guidelines to ensure compliance with legal and ethical standards in research and experimentation.