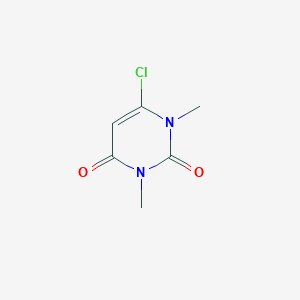
6-Chloro-1,3-dimethyluracil
Overview
Description
6-Chloro-1,3-dimethyluracil is a compound with the molecular formula C6H7ClN2O2 . It is also known by other names such as 6-chloro-1,3-dimethylpyrimidine-2,4-dione . The molecular weight of this compound is 174.58 g/mol .
Molecular Structure Analysis
The InChI code for this compound is1S/C6H7ClN2O2/c1-8-4(7)3-5(10)9(2)6(8)11/h3H,1-2H3
. The Canonical SMILES representation is CN1C(=CC(=O)N(C1=O)C)Cl
. Chemical Reactions Analysis
There is a reported acid-catalyzed photoreaction of this compound with mesitylene, leading to the formation of photocycloadducts .Physical And Chemical Properties Analysis
The compound is considered to have a molecular weight of 174.58 g/mol . Other physical and chemical properties like melting point, boiling point, etc., are not available in the resources.Scientific Research Applications
Decarboxylation and Intermediate Formation : 6-Benzyl-1,3-dimethyluracil was isolated during the decarboxylation of 1,3-dimethylorotic acid, indicating the role of a carbon-6 centered nucleophilic intermediate in the reaction pathway (Nakanishi & Wu, 1998).
Photocycloaddition Reactions : Novel cycloaddition products of the pyrimidine ring to the benzene moiety were obtained by UV irradiation of 6-chloro-1,3-dimethyluracil in xylene, demonstrating its reactivity under specific conditions (Ohkura et al., 1993).
Photoreaction in Frozen Benzene : When reacted in frozen benzene, this compound showed different behavior compared to reactions at ambient temperature, leading to the formation of novel photocycloadducts (Ohkura, Noguchi & Seki, 1997).
Photochemical Reactions in Aqueous Solutions : Kinetic analysis of photochemical reactions of 1,3-dimethyluracil in aqueous solutions revealed dependency on the substituent groups and reaction conditions (Wang, 1962).
Synthesis from 6-Chloro-5-formyl-1,3-dimethyluracil : The synthesis of 5,6-dicyano-1,3-dimethyluracil from 6-chloro-5-formyl-1,3-dimethyluracil, and its subsequent reactions, demonstrated the versatility of this compound in synthesis processes (Senda, Hirota & Asao, 1978).
Acid-Catalyzed Photocycloaddition with Aromatic Hydrocarbons : The acid-catalyzed photoreaction of this compound with various polycyclic arenes yielded cyclobutapyrimidines and 6-aryl-1,3-dimethyluracils (Seki et al., 2003).
Electronic Structure and Biological Activity : The electronic structure of this compound was analyzed using photoelectron spectroscopy, providing insights into its interaction with enzymes and potential biological activity (Novák & Kovač, 2005).
Safety and Hazards
6-Chloro-1,3-dimethyluracil is considered hazardous according to the 2012 OSHA Hazard Communication Standard (29 CFR 1910.1200). It can cause skin irritation, serious eye irritation, and may cause respiratory irritation . It is recommended to avoid breathing dust/fume/gas/mist/vapors/spray and to use only outdoors or in a well-ventilated area .
Mechanism of Action
Mode of Action
It has been reported that the compound undergoes a photocycloaddition reaction when exposed to light . This suggests that the compound may interact with its targets through a light-dependent mechanism, leading to changes in the target molecules. More detailed studies are required to fully understand this interaction.
Action Environment
The action of 6-Chloro-1,3-dimethyluracil is influenced by environmental factors such as light, given its involvement in photocycloaddition reactions . This suggests that the compound’s action, efficacy, and stability may vary depending on the light conditions.
properties
IUPAC Name |
6-chloro-1,3-dimethylpyrimidine-2,4-dione | |
---|---|---|
Source | PubChem | |
URL | https://pubchem.ncbi.nlm.nih.gov | |
Description | Data deposited in or computed by PubChem | |
InChI |
InChI=1S/C6H7ClN2O2/c1-8-4(7)3-5(10)9(2)6(8)11/h3H,1-2H3 | |
Source | PubChem | |
URL | https://pubchem.ncbi.nlm.nih.gov | |
Description | Data deposited in or computed by PubChem | |
InChI Key |
VATQPUHLFQHDBD-UHFFFAOYSA-N | |
Source | PubChem | |
URL | https://pubchem.ncbi.nlm.nih.gov | |
Description | Data deposited in or computed by PubChem | |
Canonical SMILES |
CN1C(=CC(=O)N(C1=O)C)Cl | |
Source | PubChem | |
URL | https://pubchem.ncbi.nlm.nih.gov | |
Description | Data deposited in or computed by PubChem | |
Molecular Formula |
C6H7ClN2O2 | |
Source | PubChem | |
URL | https://pubchem.ncbi.nlm.nih.gov | |
Description | Data deposited in or computed by PubChem | |
DSSTOX Substance ID |
DTXSID40220008 | |
Record name | 6-Chloro-1,3-dimethyluracil | |
Source | EPA DSSTox | |
URL | https://comptox.epa.gov/dashboard/DTXSID40220008 | |
Description | DSSTox provides a high quality public chemistry resource for supporting improved predictive toxicology. | |
Molecular Weight |
174.58 g/mol | |
Source | PubChem | |
URL | https://pubchem.ncbi.nlm.nih.gov | |
Description | Data deposited in or computed by PubChem | |
CAS RN |
6972-27-6 | |
Record name | 6-Chloro-1,3-dimethyl-2,4(1H,3H)-pyrimidinedione | |
Source | CAS Common Chemistry | |
URL | https://commonchemistry.cas.org/detail?cas_rn=6972-27-6 | |
Description | CAS Common Chemistry is an open community resource for accessing chemical information. Nearly 500,000 chemical substances from CAS REGISTRY cover areas of community interest, including common and frequently regulated chemicals, and those relevant to high school and undergraduate chemistry classes. This chemical information, curated by our expert scientists, is provided in alignment with our mission as a division of the American Chemical Society. | |
Explanation | The data from CAS Common Chemistry is provided under a CC-BY-NC 4.0 license, unless otherwise stated. | |
Record name | 6-Chloro-1,3-dimethyluracil | |
Source | ChemIDplus | |
URL | https://pubchem.ncbi.nlm.nih.gov/substance/?source=chemidplus&sourceid=0006972276 | |
Description | ChemIDplus is a free, web search system that provides access to the structure and nomenclature authority files used for the identification of chemical substances cited in National Library of Medicine (NLM) databases, including the TOXNET system. | |
Record name | 6972-27-6 | |
Source | DTP/NCI | |
URL | https://dtp.cancer.gov/dtpstandard/servlet/dwindex?searchtype=NSC&outputformat=html&searchlist=61919 | |
Description | The NCI Development Therapeutics Program (DTP) provides services and resources to the academic and private-sector research communities worldwide to facilitate the discovery and development of new cancer therapeutic agents. | |
Explanation | Unless otherwise indicated, all text within NCI products is free of copyright and may be reused without our permission. Credit the National Cancer Institute as the source. | |
Record name | 6-Chloro-1,3-dimethyluracil | |
Source | EPA DSSTox | |
URL | https://comptox.epa.gov/dashboard/DTXSID40220008 | |
Description | DSSTox provides a high quality public chemistry resource for supporting improved predictive toxicology. | |
Record name | 6-chloro-1,3-dimethyluracil | |
Source | European Chemicals Agency (ECHA) | |
URL | https://echa.europa.eu/substance-information/-/substanceinfo/100.027.460 | |
Description | The European Chemicals Agency (ECHA) is an agency of the European Union which is the driving force among regulatory authorities in implementing the EU's groundbreaking chemicals legislation for the benefit of human health and the environment as well as for innovation and competitiveness. | |
Explanation | Use of the information, documents and data from the ECHA website is subject to the terms and conditions of this Legal Notice, and subject to other binding limitations provided for under applicable law, the information, documents and data made available on the ECHA website may be reproduced, distributed and/or used, totally or in part, for non-commercial purposes provided that ECHA is acknowledged as the source: "Source: European Chemicals Agency, http://echa.europa.eu/". Such acknowledgement must be included in each copy of the material. ECHA permits and encourages organisations and individuals to create links to the ECHA website under the following cumulative conditions: Links can only be made to webpages that provide a link to the Legal Notice page. | |
Record name | 6-CHLORO-1,3-DIMETHYLURACIL | |
Source | FDA Global Substance Registration System (GSRS) | |
URL | https://gsrs.ncats.nih.gov/ginas/app/beta/substances/D2KV5G5M9F | |
Description | The FDA Global Substance Registration System (GSRS) enables the efficient and accurate exchange of information on what substances are in regulated products. Instead of relying on names, which vary across regulatory domains, countries, and regions, the GSRS knowledge base makes it possible for substances to be defined by standardized, scientific descriptions. | |
Explanation | Unless otherwise noted, the contents of the FDA website (www.fda.gov), both text and graphics, are not copyrighted. They are in the public domain and may be republished, reprinted and otherwise used freely by anyone without the need to obtain permission from FDA. Credit to the U.S. Food and Drug Administration as the source is appreciated but not required. | |
Synthesis routes and methods
Procedure details
Retrosynthesis Analysis
AI-Powered Synthesis Planning: Our tool employs the Template_relevance Pistachio, Template_relevance Bkms_metabolic, Template_relevance Pistachio_ringbreaker, Template_relevance Reaxys, Template_relevance Reaxys_biocatalysis model, leveraging a vast database of chemical reactions to predict feasible synthetic routes.
One-Step Synthesis Focus: Specifically designed for one-step synthesis, it provides concise and direct routes for your target compounds, streamlining the synthesis process.
Accurate Predictions: Utilizing the extensive PISTACHIO, BKMS_METABOLIC, PISTACHIO_RINGBREAKER, REAXYS, REAXYS_BIOCATALYSIS database, our tool offers high-accuracy predictions, reflecting the latest in chemical research and data.
Strategy Settings
Precursor scoring | Relevance Heuristic |
---|---|
Min. plausibility | 0.01 |
Model | Template_relevance |
Template Set | Pistachio/Bkms_metabolic/Pistachio_ringbreaker/Reaxys/Reaxys_biocatalysis |
Top-N result to add to graph | 6 |
Feasible Synthetic Routes
Q & A
Q1: What is the molecular formula and weight of 6-Chloro-1,3-dimethyluracil?
A1: The molecular formula of this compound is C6H7ClN2O2, and its molecular weight is 174.59 g/mol.
Q2: Is there any spectroscopic data available for this compound?
A2: While the provided abstracts do not detail specific spectroscopic data, researchers often characterize this compound using techniques such as NMR (Nuclear Magnetic Resonance) and IR (Infrared) spectroscopy to confirm its structure and analyze its properties.
Q3: How is this compound synthesized?
A3: One method involves refluxing 1,3-dimethylbarbituric acid with a chlorinating agent, such as phosphorus oxychloride, in a water-insoluble organic solvent []. Another approach uses 6-azido-1,3-dimethyluracil reacted with acyl halides under irradiation [].
Q4: Can you elaborate on the photochemical reactions of this compound?
A4: This compound exhibits interesting photochemical behavior. For example, it undergoes acid-catalyzed photocycloaddition with various substrates. This includes reactions with polycyclic aromatic hydrocarbons like phenanthrene and pyrene, yielding cyclobutapyrimidines []. It also reacts with p- and m-xylene, forming novel diazapentacyclododecane derivatives [].
Q5: How does this compound react with naphthalene under UV irradiation?
A7: The reaction outcome depends on the solvent polarity. In non-polar solvents, a substitution reaction produces 6-(1-naphthyluracil). Conversely, in polar media, a 1,2-cycloaddition occurs, generating naphthocyclobutapyrimidines. Notably, adding trifluoroacetic acid (TFA) can also promote the cycloaddition in non-polar solvents [].
Q6: Are there any significant reactions involving dimethylsulfoxonium methylide?
A8: Reacting this compound with two equivalents of dimethylsulfoxonium methylide forms a sulfoxonium ylide []. This ylide shows versatile reactivity, undergoing deuterium exchange and engaging in reactions with electrophiles like benzoyl chloride and electron-deficient olefins, ultimately leading to various substituted uracil derivatives.
Q7: What are the potential applications of this compound?
A9: The research primarily focuses on its synthetic utility as a building block for complex heterocyclic systems. These complex structures hold potential as pharmaceutical agents or biological probes. For instance, a study explored its use in synthesizing derivatives of 3-(β-D-ribofuranosyl)uracil, which could potentially act as inhibitors for orotidine 5’-monophosphate decarboxylase (ODCase) [].
Disclaimer and Information on In-Vitro Research Products
Please be aware that all articles and product information presented on BenchChem are intended solely for informational purposes. The products available for purchase on BenchChem are specifically designed for in-vitro studies, which are conducted outside of living organisms. In-vitro studies, derived from the Latin term "in glass," involve experiments performed in controlled laboratory settings using cells or tissues. It is important to note that these products are not categorized as medicines or drugs, and they have not received approval from the FDA for the prevention, treatment, or cure of any medical condition, ailment, or disease. We must emphasize that any form of bodily introduction of these products into humans or animals is strictly prohibited by law. It is essential to adhere to these guidelines to ensure compliance with legal and ethical standards in research and experimentation.