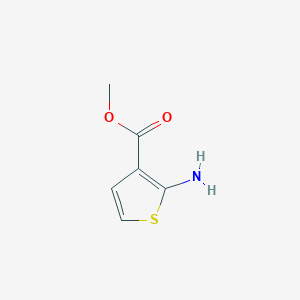
Methyl 2-aminothiophene-3-carboxylate
Overview
Description
Methyl 2-aminothiophene-3-carboxylate (CAS 4651-81-4) is a heterocyclic compound featuring a thiophene ring substituted with an amino group at position 2 and a methyl ester at position 2. This scaffold is widely utilized in medicinal chemistry and materials science due to its electronic properties and versatility in synthesis. It serves as a key intermediate in the development of bioactive molecules, including kinase inhibitors (e.g., PI3Kα inhibitors) , antimicrobial agents , and organic semiconductors . The compound is typically synthesized via the Gewald reaction, a one-pot condensation of ketones, cyanoacetates, and sulfur , or obtained commercially for further derivatization. Its reactivity, particularly in N-arylation and cyclization reactions, makes it a cornerstone for constructing complex heterocyclic systems like thieno[2,3-d]pyrimidines .
Preparation Methods
Gewald Reaction: Cyclocondensation of Methyl Cyanoacetate
The Gewald reaction remains the most widely employed method for synthesizing methyl 2-aminothiophene-3-carboxylate. This one-pot, three-component reaction involves methyl cyanoacetate, a ketone, and elemental sulfur, catalyzed by a base such as morpholine .
Reaction Mechanism and Conditions
The reaction proceeds via a stepwise mechanism:
-
Knoevenagel Condensation : Methyl cyanoacetate reacts with a ketone (e.g., cyclohexanone) to form an α,β-unsaturated nitrile intermediate.
-
Sulfur Incorporation : Elemental sulfur undergoes nucleophilic attack by the enolate of the nitrile, forming a thiiranium intermediate.
-
Cyclization and Aromatization : Intramolecular cyclization yields the thiophene ring, followed by oxidation to the aromatic structure .
Key parameters influencing yield and purity include:
-
Catalyst : Morpholine (5–10 mol%) optimally facilitates both condensation and cyclization .
-
Temperature : Reactions proceed at 35–45°C, with prolonged stirring (3–5 hours) ensuring completion .
-
Solvent : Methanol or ethanol is preferred for solubility and ease of workup .
Table 1: Gewald Reaction Optimization Data
Ketone | Catalyst | Temp (°C) | Time (h) | Yield (%) |
---|---|---|---|---|
Cyclohexanone | Morpholine | 40 | 3 | 78–82 |
Acetophenone | Morpholine | 45 | 4 | 70–75 |
Cyclopentanone | Morpholine | 35 | 3.5 | 80–85 |
Yields typically range from 70% to 85% after recrystallization from ethanol . The method tolerates diverse ketones, including aliphatic and aryl-substituted variants, though steric hindrance in bulky ketones may reduce efficiency .
Alternative Synthesis via Dihalo Nitriles and Thioglycolate Esters
A patent-published method (DE1055007B) employs α,β-dihalo nitriles and thioglycolic acid esters under alkaline conditions . This route offers distinct advantages for scale-up and functional group compatibility.
Procedure and Optimization
-
Reactants : α,β-Dichloroacrylonitrile reacts with methyl thioglycolate (HS-CH₂COOCH₃) in methanol.
-
Base : Sodium methoxide (2 equiv) deprotonates the thiol, enabling nucleophilic substitution.
-
Cyclization : Spontaneous cyclization forms the thiophene core, followed by amination via nitrile hydrolysis .
Critical factors:
-
Solvent : Methanol balances reactivity and solubility, preventing side reactions.
-
Temperature : Room temperature (20–25°C) suffices, minimizing decomposition.
-
Workup : Acidification with HCl precipitates the product, yielding 65–75% purity before recrystallization .
Table 2: Dihalo Nitrile Route Performance
Dihalo Nitrile | Thioglycolate Ester | Base | Yield (%) |
---|---|---|---|
α,β-Dichloroacrylonitrile | Methyl thioglycolate | NaOMe | 68 |
α,β-Dibromoacrylonitrile | Ethyl thioglycolate | KOtBu | 72 |
This method avoids sulfur handling but requires stringent control of nitrile hydrolysis to prevent over-amination .
Modified Gewald Approaches for Functionalized Derivatives
Recent adaptations of the Gewald reaction enable direct incorporation of pharmacophores. For example, 3-thiaisatoic anhydride —a derivative of this compound—is synthesized via hydrolysis followed by phosgene treatment .
Hydrolysis and Anhydride Formation
-
Hydrolysis : Lithium hydroxide (2 equiv) in THF/water (3:1) cleaves the methyl ester to 2-aminothiophene-3-carboxylic acid (85–90% yield) .
-
Phosgene Cyclization : Treatment with phosgene (1.2 equiv) in dichloromethane forms the anhydride (75–80% yield) .
This two-step process highlights the compound’s versatility as a building block for heterocyclic systems .
Comparative Analysis of Synthetic Routes
Table 3: Method Comparison
Parameter | Gewald Reaction | Dihalo Nitrile Route | Hydrolysis/Anhydride |
---|---|---|---|
Yield (%) | 70–85 | 65–75 | 75–80 |
Scalability | High | Moderate | Low |
Functional Tolerance | Broad | Limited | Narrow |
Cost | Low | Moderate | High |
The Gewald reaction excels in cost and scalability, making it ideal for industrial applications. Conversely, the dihalo nitrile route suits laboratories with nitrile-handling infrastructure .
Chemical Reactions Analysis
Oxidation Reactions
MATC undergoes oxidation to form sulfoxides and sulfones. Typical oxidizing agents include hydrogen peroxide (H₂O₂) and meta-chloroperbenzoic acid (mCPBA):
The sulfone derivatives exhibit enhanced stability and are used in medicinal chemistry for antiviral and anticancer applications .
Reduction Reactions
Reduction of MATC’s ester group yields thiophene alcohols or amines:
Reducing Agent | Conditions | Products | Yield | Reference |
---|---|---|---|---|
LiAlH₄ | THF, 0°C → rt, 3h | 2-Aminothiophene-3-methanol | 82% | |
NaBH₄/CuCl₂ | Methanol, rt, 6h | 2-Amino-3-hydroxymethylthiophene | 58% |
These reduced products serve as intermediates for polymer synthesis and ligand design .
Nucleophilic Substitution Reactions
The amino group in MATC participates in nucleophilic substitutions with alkyl halides, acyl chlorides, and sulfonyl chlorides:
Substituted derivatives are pivotal in developing kinase inhibitors and antimicrobial agents .
Chan-Lam Coupling for N-Arylation
Copper-mediated Chan-Lam coupling enables N-arylation using arylboronic acids or trifluoroborates:
Arylating Agent | Catalyst | Conditions | Yield | Reference |
---|---|---|---|---|
Phenylboronic acid | Cu(OAc)₂, Et₃N, CH₂Cl₂ | rt, 12h, air | 68% | |
4-NO₂-C₆H₄BF₃K | Cu(OAc)₂, DMAP, DCE | 50°C, 24h, O₂ | 73% |
This method tolerates electron-withdrawing and donating groups, enabling access to diverse N-aryl derivatives for drug discovery .
Cyclocondensation Reactions
MATC participates in Gewald-type cyclocondensation with aldehydes and ketones to form polycyclic thiophenes:
These products are precursors for fluorescent dyes and photovoltaic materials .
Sulfur-Based Modifications
Reactions with sulfur reagents enable sulfenylation or thiolation:
Thiolated derivatives show promise in chelating heavy metals and radical scavenging .
Ester Hydrolysis and Functionalization
The methyl ester group undergoes hydrolysis to carboxylic acids or transesterification:
Reagent | Conditions | Products | Yield | Reference |
---|---|---|---|---|
LiOH | THF/H₂O, rt, 4h | 2-Aminothiophene-3-carboxylic acid | 95% | |
Ethanol/H₂SO₄ | Reflux, 8h | Ethyl 2-aminothiophene-3-carboxylate | 88% |
The carboxylic acid derivative is a key intermediate for peptide-conjugated therapeutics .
Key Mechanistic Insights
- Intramolecular hydrogen bonding between the amino and ester groups (N–H⋯O=C) stabilizes intermediates during substitution and coupling reactions .
- Copper coordination in Chan-Lam coupling involves a Cu(II)/Cu(III) cycle, facilitating aryl transfer to the amino group .
- Solvent polarity influences reaction pathways; polar aprotic solvents (DMF, DCE) favor N-arylation, while protic solvents (MeOH) enhance ester hydrolysis .
This comprehensive analysis underscores MATC’s utility in synthesizing bioactive molecules and advanced materials.
Scientific Research Applications
Organic Synthesis
MATC serves as a crucial building block in the synthesis of complex organic molecules. Its derivatives are utilized in constructing heterocyclic compounds, particularly thieno[2,3-d]pyrimidine derivatives, which have shown promise in various biological activities.
Key Synthetic Routes:
- Reaction with thiophene-2-carboxylic acid to form methyl ester.
- Aminolysis with ammonia or amines to yield derivatives.
- Copper-mediated N-arylation reactions with organoboron reagents for functionalization .
Medicinal Chemistry
MATC has been studied extensively for its potential therapeutic applications. It exhibits activity against multiple drug-resistant strains of Mycobacterium tuberculosis (Mtb), highlighting its significance in developing anti-tuberculosis agents.
Case Study:
A study evaluated a series of 2-aminothiophenes, including MATC, against Mtb. Compounds derived from MATC demonstrated low minimum inhibitory concentrations (MICs), indicating their potential as effective antimycobacterial agents .
Compound | MIC (μg/mL) | Selectivity Index |
---|---|---|
MATC Derivative 1 | 0.69 | >10 |
MATC Derivative 2 | 0.03 | >3200 |
MATC Derivative 3 | 0.02 | >2000 |
Biochemical Studies
In biochemical research, MATC is employed as a probe to study enzyme interactions and cellular processes. It has been shown to modulate the activity of key enzymes involved in cell signaling pathways.
Mechanism of Action:
MATC targets enzymes such as phosphatidylinositol 3-kinase (PI3K), influencing pathways related to cell proliferation and apoptosis. Its ability to disrupt normal cellular processes makes it a candidate for anticancer therapies .
Dyes and Pigments
MATC is utilized in the synthesis of dyes due to its chromophoric properties. The compound's derivatives can be engineered to produce vibrant colors suitable for various industrial applications.
Pesticides
The compound has also found applications in agrochemicals, specifically as an intermediate in the synthesis of pesticides. Its efficacy against pests contributes to its relevance in agricultural chemistry.
Mechanism of Action
The mechanism of action of methyl 2-aminothiophene-3-carboxylate involves its interaction with specific molecular targets and pathways. The amino and carboxyl groups in the compound can participate in various inter- and intra-molecular interactions, influencing its reactivity and biological activity. For instance, in pharmaceutical applications, the compound may inhibit specific enzymes or receptors, leading to therapeutic effects .
Comparison with Similar Compounds
Methyl 2-aminothiophene-3-carboxylate belongs to a family of 2-aminothiophene-3-carboxylate esters. Below is a detailed comparison with structurally related analogs, focusing on synthesis, reactivity, and applications.
Structural and Functional Variations
Physicochemical Properties
- Solubility: Methyl and ethyl esters exhibit moderate solubility in polar solvents (e.g., ethanol, THF), while tert-butyl derivatives are more hydrophobic.
- Stability : tert-Boc protection enhances stability under acidic conditions, making it suitable for multi-step syntheses .
Research Findings and Trends
- Chan-Lam Coupling: this compound’s compatibility with aryltrifluoroborates expands access to diverse N-aryl derivatives under ambient conditions .
- Thienopyrimidine Scaffolds: Cyclization reactions of methyl/ethyl esters yield fused heterocycles with broad bioactivity, driving innovation in kinase inhibitor design .
- SAR Insights : Substituents on the thiophene ring (e.g., 4-methyl, 5-isopropyl) fine-tune electronic and steric effects, optimizing drug-target interactions .
Biological Activity
Methyl 2-aminothiophene-3-carboxylate (MATC) is an organic compound with significant biological activity, particularly in the fields of medicinal chemistry and pharmacology. This article explores its biological properties, mechanisms of action, and potential therapeutic applications, supported by relevant data and case studies.
Chemical Structure and Properties
Chemical Formula: C₆H₇NO₂S
CAS Number: 4651-81-4
MATC is characterized by its thiophene ring, amino group, and carboxylate functionality, which contribute to its reactivity and biological interactions. The compound's ability to form hydrogen bonds and engage in electrostatic interactions enhances its potential as a bioactive molecule.
MATC primarily targets key enzymes and receptors involved in various biochemical pathways. Notably, it interacts with:
- Phosphatidylinositol 3-kinase (PI3K) : Influencing cell proliferation and survival.
- DprE1 (Decaprenyl-phosphoryl-β-D-ribose 2'-oxidase) : A critical enzyme in the mycobacterial cell wall biosynthesis pathway.
Interaction Dynamics
The amino and carboxyl groups of MATC facilitate binding to target proteins through:
- Hydrogen bonding
- Electrostatic interactions
These interactions can lead to the modulation or inhibition of enzyme activity, impacting cellular functions such as apoptosis and viral replication cycles.
Antimicrobial Properties
MATC has shown promising activity against Mycobacterium tuberculosis (Mtb). Studies indicate that derivatives of MATC exhibit low minimum inhibitory concentrations (MIC), demonstrating potent antimycobacterial effects. For instance:
Compound | MIC (μg/mL) | Selectivity Index (SI) |
---|---|---|
23j | 0.02 | >3200 |
25b | 0.03 | >2133 |
These compounds not only inhibit drug-susceptible strains but also show efficacy against multidrug-resistant strains of Mtb, highlighting their therapeutic potential in treating tuberculosis .
Anticancer Activity
Research has demonstrated that MATC derivatives possess cytostatic properties, selectively inducing apoptosis in certain cancer cell lines while sparing non-tumorigenic cells. For example:
- In prostate cancer models, MATC derivatives led to cell cycle arrest in the G1 phase and reduced protein synthesis preferentially .
The following table summarizes the cytotoxic effects observed in various cancer cell lines:
Cell Line | IC50 (nM) | Effect |
---|---|---|
Prostate Cancer | ~20 | Induces apoptosis |
T-cell Lymphoma | ~50 | Cytostatic activity |
Kidney Carcinoma | ~40 | Selective suppression |
Pharmacokinetics
The pharmacokinetic profile of MATC includes:
- Absorption : Efficiently absorbed through the gastrointestinal tract.
- Distribution : Widely distributed within body tissues.
- Metabolism : Primarily metabolized in the liver.
- Excretion : Eliminated via renal pathways.
These properties influence MATC's bioavailability and therapeutic efficacy .
Case Study 1: Antimycobacterial Activity
In a study evaluating novel thiophene-arylamide derivatives based on MATC, compounds were tested for their ability to inhibit Mtb growth. The results indicated that modifications to the MATC structure significantly enhanced potency against both drug-susceptible and resistant strains .
Case Study 2: Cancer Cell Line Selectivity
A series of experiments assessed the cytotoxic effects of MATC derivatives on various cancer cell lines. The findings revealed a marked selectivity for T-cell lymphoma cells over B-cell lymphoma cells, suggesting that MATC derivatives could be developed into targeted therapies for specific cancer types .
Q & A
Basic Research Questions
Q. What are the optimized synthetic routes for Methyl 2-aminothiophene-3-carboxylate, and what challenges arise during its preparation?
- Methodology : The Gewald reaction is the most common method, involving a one-pot reaction of ethyl cyanoacetate, elemental sulfur, and an aldehyde/ketone under basic conditions. For example, synthesis of derivatives via this route achieved yields of 60–80% . Key challenges include controlling regioselectivity and avoiding side reactions (e.g., over-oxidation).
- Troubleshooting : Use anhydrous solvents (e.g., DMF or ethanol) and monitor reaction progress via TLC. Post-reaction purification via column chromatography (silica gel, CH₂Cl₂/MeOH) or recrystallization improves purity .
Q. How can the structure of this compound derivatives be confirmed experimentally?
- Analytical Techniques :
- NMR : Key signals include NH₂ protons (δ 6.5–7.0 ppm, broad singlet) and thiophene ring protons (δ 6.8–7.5 ppm) .
- IR : Stretching vibrations for NH₂ (~3350 cm⁻¹), ester C=O (~1700 cm⁻¹), and C-O (~1250 cm⁻¹) confirm functional groups .
- Melting Point : Pure derivatives typically melt between 76–226°C, depending on substituents .
Q. What are the common functionalization strategies for this compound?
- Reactions :
- Amide Coupling : React with acyl chlorides (e.g., benzoyl chloride) in THF/TEA to form 2-benzamidothiophene derivatives .
- N-Arylation : Copper-mediated coupling with arylboronic acids under aerobic conditions introduces aryl groups at the amino position .
- Hydrolysis : Convert the ester to a carboxylic acid using NaOH/EtOH for further derivatization .
Advanced Research Questions
Q. How can computational modeling guide the design of this compound-based inhibitors?
- Approach : Density Functional Theory (DFT) calculations predict electronic properties (e.g., HOMO-LUMO gaps) to optimize interactions with target proteins like PI3Kα. Crystal structure data (e.g., puckering coordinates from Cremer-Pople analysis) inform conformational stability .
- Case Study : Thienopyrimidine derivatives showed enhanced PI3Kα inhibition (IC₅₀ = 0.8–12 nM) when substituents were optimized for hydrogen bonding with ATP-binding pockets .
Q. What strategies resolve contradictions in biological activity data for structurally similar derivatives?
- Data Analysis :
-
Compare IC₅₀ values across assays (e.g., enzymatic vs. cellular) to identify off-target effects.
-
Use SAR tables to correlate substituent electronegativity/logP with activity (Table 1).
Table 1 : Example SAR for PI3Kα Inhibitors
Substituent (R) LogP IC₅₀ (nM) -OCH₃ 1.2 0.8 -CF₃ 2.5 12.0 -Cl 1.8 5.4
Q. How are crystallographic data used to validate the 3D structure of this compound derivatives?
- Techniques : Single-crystal X-ray diffraction (SHELX refinement) confirms bond lengths (C-S: ~1.70 Å) and ring puckering parameters (e.g., Cremer-Pople coordinates for non-planar conformations) .
- Software : ORTEP-3 and WinGX generate thermal ellipsoid plots and electron density maps .
Q. What are the challenges in achieving regioselective modifications on the thiophene ring?
- Mechanistic Insight : Electron-withdrawing groups (e.g., -COOCH₃) direct electrophilic substitution to the 5-position. For example, hydroformylation of trifluoromethyl-substituted derivatives shows >90% regioselectivity under Rh catalysis .
- Optimization : Use Lewis acids (e.g., BF₃·Et₂O) to enhance electrophilic character or protect reactive sites with Boc groups .
Q. Methodological Recommendations
- Synthesis : Prioritize the Gewald reaction for scalability and Chan-Lam coupling for N-arylation .
- Purification : Employ reverse-phase HPLC (MeOH/H₂O gradient) for polar derivatives .
- Data Validation : Cross-reference NMR/IR with computational predictions (e.g., Gaussian 09) to confirm assignments .
Properties
IUPAC Name |
methyl 2-aminothiophene-3-carboxylate | |
---|---|---|
Source | PubChem | |
URL | https://pubchem.ncbi.nlm.nih.gov | |
Description | Data deposited in or computed by PubChem | |
InChI |
InChI=1S/C6H7NO2S/c1-9-6(8)4-2-3-10-5(4)7/h2-3H,7H2,1H3 | |
Source | PubChem | |
URL | https://pubchem.ncbi.nlm.nih.gov | |
Description | Data deposited in or computed by PubChem | |
InChI Key |
DGGJQLCAYQCPDD-UHFFFAOYSA-N | |
Source | PubChem | |
URL | https://pubchem.ncbi.nlm.nih.gov | |
Description | Data deposited in or computed by PubChem | |
Canonical SMILES |
COC(=O)C1=C(SC=C1)N | |
Source | PubChem | |
URL | https://pubchem.ncbi.nlm.nih.gov | |
Description | Data deposited in or computed by PubChem | |
Molecular Formula |
C6H7NO2S | |
Source | PubChem | |
URL | https://pubchem.ncbi.nlm.nih.gov | |
Description | Data deposited in or computed by PubChem | |
DSSTOX Substance ID |
DTXSID70196863 | |
Record name | Methyl 2-amino-3-thenoate | |
Source | EPA DSSTox | |
URL | https://comptox.epa.gov/dashboard/DTXSID70196863 | |
Description | DSSTox provides a high quality public chemistry resource for supporting improved predictive toxicology. | |
Molecular Weight |
157.19 g/mol | |
Source | PubChem | |
URL | https://pubchem.ncbi.nlm.nih.gov | |
Description | Data deposited in or computed by PubChem | |
CAS No. |
4651-81-4 | |
Record name | Methyl 2-amino-3-thiophenecarboxylate | |
Source | CAS Common Chemistry | |
URL | https://commonchemistry.cas.org/detail?cas_rn=4651-81-4 | |
Description | CAS Common Chemistry is an open community resource for accessing chemical information. Nearly 500,000 chemical substances from CAS REGISTRY cover areas of community interest, including common and frequently regulated chemicals, and those relevant to high school and undergraduate chemistry classes. This chemical information, curated by our expert scientists, is provided in alignment with our mission as a division of the American Chemical Society. | |
Explanation | The data from CAS Common Chemistry is provided under a CC-BY-NC 4.0 license, unless otherwise stated. | |
Record name | Methyl 2-amino-3-thenoate | |
Source | ChemIDplus | |
URL | https://pubchem.ncbi.nlm.nih.gov/substance/?source=chemidplus&sourceid=0004651814 | |
Description | ChemIDplus is a free, web search system that provides access to the structure and nomenclature authority files used for the identification of chemical substances cited in National Library of Medicine (NLM) databases, including the TOXNET system. | |
Record name | 4651-81-4 | |
Source | DTP/NCI | |
URL | https://dtp.cancer.gov/dtpstandard/servlet/dwindex?searchtype=NSC&outputformat=html&searchlist=523742 | |
Description | The NCI Development Therapeutics Program (DTP) provides services and resources to the academic and private-sector research communities worldwide to facilitate the discovery and development of new cancer therapeutic agents. | |
Explanation | Unless otherwise indicated, all text within NCI products is free of copyright and may be reused without our permission. Credit the National Cancer Institute as the source. | |
Record name | Methyl 2-amino-3-thenoate | |
Source | EPA DSSTox | |
URL | https://comptox.epa.gov/dashboard/DTXSID70196863 | |
Description | DSSTox provides a high quality public chemistry resource for supporting improved predictive toxicology. | |
Record name | Methyl 2-amino-3-thenoate | |
Source | European Chemicals Agency (ECHA) | |
URL | https://echa.europa.eu/substance-information/-/substanceinfo/100.022.804 | |
Description | The European Chemicals Agency (ECHA) is an agency of the European Union which is the driving force among regulatory authorities in implementing the EU's groundbreaking chemicals legislation for the benefit of human health and the environment as well as for innovation and competitiveness. | |
Explanation | Use of the information, documents and data from the ECHA website is subject to the terms and conditions of this Legal Notice, and subject to other binding limitations provided for under applicable law, the information, documents and data made available on the ECHA website may be reproduced, distributed and/or used, totally or in part, for non-commercial purposes provided that ECHA is acknowledged as the source: "Source: European Chemicals Agency, http://echa.europa.eu/". Such acknowledgement must be included in each copy of the material. ECHA permits and encourages organisations and individuals to create links to the ECHA website under the following cumulative conditions: Links can only be made to webpages that provide a link to the Legal Notice page. | |
Record name | Methyl 2-amino-3-thenoate | |
Source | FDA Global Substance Registration System (GSRS) | |
URL | https://gsrs.ncats.nih.gov/ginas/app/beta/substances/C26DR8VS6P | |
Description | The FDA Global Substance Registration System (GSRS) enables the efficient and accurate exchange of information on what substances are in regulated products. Instead of relying on names, which vary across regulatory domains, countries, and regions, the GSRS knowledge base makes it possible for substances to be defined by standardized, scientific descriptions. | |
Explanation | Unless otherwise noted, the contents of the FDA website (www.fda.gov), both text and graphics, are not copyrighted. They are in the public domain and may be republished, reprinted and otherwise used freely by anyone without the need to obtain permission from FDA. Credit to the U.S. Food and Drug Administration as the source is appreciated but not required. | |
Synthesis routes and methods
Procedure details
Retrosynthesis Analysis
AI-Powered Synthesis Planning: Our tool employs the Template_relevance Pistachio, Template_relevance Bkms_metabolic, Template_relevance Pistachio_ringbreaker, Template_relevance Reaxys, Template_relevance Reaxys_biocatalysis model, leveraging a vast database of chemical reactions to predict feasible synthetic routes.
One-Step Synthesis Focus: Specifically designed for one-step synthesis, it provides concise and direct routes for your target compounds, streamlining the synthesis process.
Accurate Predictions: Utilizing the extensive PISTACHIO, BKMS_METABOLIC, PISTACHIO_RINGBREAKER, REAXYS, REAXYS_BIOCATALYSIS database, our tool offers high-accuracy predictions, reflecting the latest in chemical research and data.
Strategy Settings
Precursor scoring | Relevance Heuristic |
---|---|
Min. plausibility | 0.01 |
Model | Template_relevance |
Template Set | Pistachio/Bkms_metabolic/Pistachio_ringbreaker/Reaxys/Reaxys_biocatalysis |
Top-N result to add to graph | 6 |
Feasible Synthetic Routes
Disclaimer and Information on In-Vitro Research Products
Please be aware that all articles and product information presented on BenchChem are intended solely for informational purposes. The products available for purchase on BenchChem are specifically designed for in-vitro studies, which are conducted outside of living organisms. In-vitro studies, derived from the Latin term "in glass," involve experiments performed in controlled laboratory settings using cells or tissues. It is important to note that these products are not categorized as medicines or drugs, and they have not received approval from the FDA for the prevention, treatment, or cure of any medical condition, ailment, or disease. We must emphasize that any form of bodily introduction of these products into humans or animals is strictly prohibited by law. It is essential to adhere to these guidelines to ensure compliance with legal and ethical standards in research and experimentation.