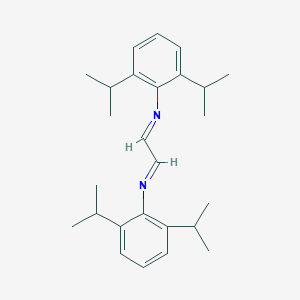
N,N'-Bis(2,6-diisopropylphenyl)ethanediimine
Overview
Description
N,N’-Bis(2,6-diisopropylphenyl)ethanediimine is a chemical compound with the molecular formula C26H40N2. It is known for its applications in organic synthesis and as an intermediate in various chemical reactions. The compound is characterized by its two diisopropylphenyl groups attached to an ethanediimine backbone, making it a valuable reagent in both academic and industrial research .
Preparation Methods
Synthetic Routes and Reaction Conditions
N,N’-Bis(2,6-diisopropylphenyl)ethanediimine can be synthesized through a reaction involving 2,6-diisopropylaniline and glyoxal. The reaction typically occurs under controlled conditions, often in the presence of a catalyst to facilitate the formation of the diimine structure . The reaction conditions include maintaining a specific temperature range and using solvents that support the reaction’s progress.
Industrial Production Methods
In industrial settings, the production of N,N’-Bis(2,6-diisopropylphenyl)ethanediimine involves scaling up the laboratory synthesis methods. This includes optimizing reaction conditions to ensure high yield and purity. The use of continuous flow reactors and advanced purification techniques, such as crystallization and chromatography, are common in industrial production to achieve the desired product quality .
Chemical Reactions Analysis
Types of Reactions
N,N’-Bis(2,6-diisopropylphenyl)ethanediimine undergoes various chemical reactions, including:
Oxidation: The compound can be oxidized to form corresponding imine oxides.
Reduction: It can be reduced to form amines.
Substitution: The diimine groups can participate in substitution reactions, where one or both of the phenyl groups are replaced by other substituents
Common Reagents and Conditions
Common reagents used in these reactions include oxidizing agents like hydrogen peroxide for oxidation, reducing agents such as lithium aluminum hydride for reduction, and various nucleophiles for substitution reactions. The conditions for these reactions vary but often involve specific temperatures, solvents, and catalysts to achieve the desired transformations .
Major Products Formed
The major products formed from these reactions depend on the type of reaction and the reagents used. For example, oxidation of N,N’-Bis(2,6-diisopropylphenyl)ethanediimine typically yields imine oxides, while reduction results in the formation of amines. Substitution reactions can produce a variety of substituted diimines depending on the nucleophiles involved .
Scientific Research Applications
N,N’-Bis(2,6-diisopropylphenyl)ethanediimine has several scientific research applications:
Chemistry: It is used as a ligand in coordination chemistry and as a reagent in organic synthesis.
Biology: The compound is studied for its potential biological activities and interactions with biomolecules.
Medicine: Research is ongoing to explore its potential therapeutic applications, including its use as a precursor for drug development.
Industry: It is used in the production of advanced materials and as an intermediate in the synthesis of various industrial chemicals
Mechanism of Action
The mechanism of action of N,N’-Bis(2,6-diisopropylphenyl)ethanediimine involves its ability to form stable complexes with metal ions, making it a valuable ligand in coordination chemistry. The compound’s diimine groups can coordinate with metal centers, facilitating various catalytic processes. Additionally, its interactions with biological molecules are being studied to understand its potential therapeutic effects .
Comparison with Similar Compounds
Similar Compounds
- N,N’-Bis(2,6-diisopropylphenyl)-1,4-diazabutadiene
- Glyoxal bis(2,6-diisopropylanil)
- N,N’-Bis(2,6-diisopropylphenyl)ethylenediamine
Uniqueness
N,N’-Bis(2,6-diisopropylphenyl)ethanediimine is unique due to its specific diimine structure, which provides distinct reactivity and coordination properties compared to similar compounds. Its bulky diisopropylphenyl groups also contribute to its stability and steric effects, making it a valuable reagent in various chemical reactions .
Biological Activity
N,N'-Bis(2,6-diisopropylphenyl)ethanediimine, a compound with the molecular formula C26H40N2, is gaining attention for its potential biological activities and applications in medicinal chemistry. This article delves into its biological activity, mechanisms of action, and relevant research findings.
This compound can be synthesized through the reaction of 2,6-diisopropylaniline with glyoxal. This reaction typically requires controlled conditions and may involve catalysts to enhance yield and purity. The compound features two bulky diisopropylphenyl groups that contribute to its stability and unique reactivity profile.
The biological activity of this compound is primarily attributed to its ability to form stable complexes with metal ions, which enhances its role as a ligand in coordination chemistry. This property allows it to participate in various catalytic processes that are essential for biological functions. Furthermore, its interactions with biomolecules are being explored for therapeutic potential.
Antimicrobial Activity
Research has indicated that this compound exhibits antimicrobial properties. A study demonstrated its effectiveness against various bacterial strains, suggesting potential applications in developing antimicrobial agents. The compound's structure allows it to interact with bacterial cell membranes, disrupting their integrity and leading to cell death.
Cytotoxic Effects
In vitro studies have shown that this compound has cytotoxic effects on cancer cell lines. For instance, it was observed to inhibit the proliferation of human tumor cells with an IC50 value indicating significant potency. These findings highlight its potential as a lead compound for anticancer drug development.
Case Studies
Study | Findings |
---|---|
Antimicrobial Activity | Demonstrated effectiveness against Gram-positive and Gram-negative bacteria, indicating broad-spectrum antimicrobial properties. |
Cytotoxicity | Inhibition of tumor cell growth in various cancer cell lines; specific IC50 values show strong anticancer potential. |
Metal Complexation | Formation of complexes with transition metals enhances catalytic activity in organic transformations relevant to drug synthesis. |
Research Applications
This compound has diverse applications in scientific research:
- Coordination Chemistry : It serves as a ligand for metal complexes used in catalysis.
- Drug Development : Investigated for its potential as a precursor in synthesizing new therapeutic agents.
- Material Science : Utilized in creating advanced materials due to its unique chemical properties.
Properties
IUPAC Name |
N,N'-bis[2,6-di(propan-2-yl)phenyl]ethane-1,2-diimine | |
---|---|---|
Source | PubChem | |
URL | https://pubchem.ncbi.nlm.nih.gov | |
Description | Data deposited in or computed by PubChem | |
InChI |
InChI=1S/C26H36N2/c1-17(2)21-11-9-12-22(18(3)4)25(21)27-15-16-28-26-23(19(5)6)13-10-14-24(26)20(7)8/h9-20H,1-8H3 | |
Source | PubChem | |
URL | https://pubchem.ncbi.nlm.nih.gov | |
Description | Data deposited in or computed by PubChem | |
InChI Key |
JWVIIGXMTONOFR-UHFFFAOYSA-N | |
Source | PubChem | |
URL | https://pubchem.ncbi.nlm.nih.gov | |
Description | Data deposited in or computed by PubChem | |
Canonical SMILES |
CC(C)C1=C(C(=CC=C1)C(C)C)N=CC=NC2=C(C=CC=C2C(C)C)C(C)C | |
Source | PubChem | |
URL | https://pubchem.ncbi.nlm.nih.gov | |
Description | Data deposited in or computed by PubChem | |
Molecular Formula |
C26H36N2 | |
Source | PubChem | |
URL | https://pubchem.ncbi.nlm.nih.gov | |
Description | Data deposited in or computed by PubChem | |
DSSTOX Substance ID |
DTXSID00348116 | |
Record name | (1E,2E)-N~1~,N~2~-Bis[2,6-di(propan-2-yl)phenyl]ethane-1,2-diimine | |
Source | EPA DSSTox | |
URL | https://comptox.epa.gov/dashboard/DTXSID00348116 | |
Description | DSSTox provides a high quality public chemistry resource for supporting improved predictive toxicology. | |
Molecular Weight |
376.6 g/mol | |
Source | PubChem | |
URL | https://pubchem.ncbi.nlm.nih.gov | |
Description | Data deposited in or computed by PubChem | |
CAS No. |
74663-75-5 | |
Record name | (1E,2E)-N~1~,N~2~-Bis[2,6-di(propan-2-yl)phenyl]ethane-1,2-diimine | |
Source | EPA DSSTox | |
URL | https://comptox.epa.gov/dashboard/DTXSID00348116 | |
Description | DSSTox provides a high quality public chemistry resource for supporting improved predictive toxicology. | |
Retrosynthesis Analysis
AI-Powered Synthesis Planning: Our tool employs the Template_relevance Pistachio, Template_relevance Bkms_metabolic, Template_relevance Pistachio_ringbreaker, Template_relevance Reaxys, Template_relevance Reaxys_biocatalysis model, leveraging a vast database of chemical reactions to predict feasible synthetic routes.
One-Step Synthesis Focus: Specifically designed for one-step synthesis, it provides concise and direct routes for your target compounds, streamlining the synthesis process.
Accurate Predictions: Utilizing the extensive PISTACHIO, BKMS_METABOLIC, PISTACHIO_RINGBREAKER, REAXYS, REAXYS_BIOCATALYSIS database, our tool offers high-accuracy predictions, reflecting the latest in chemical research and data.
Strategy Settings
Precursor scoring | Relevance Heuristic |
---|---|
Min. plausibility | 0.01 |
Model | Template_relevance |
Template Set | Pistachio/Bkms_metabolic/Pistachio_ringbreaker/Reaxys/Reaxys_biocatalysis |
Top-N result to add to graph | 6 |
Feasible Synthetic Routes
Disclaimer and Information on In-Vitro Research Products
Please be aware that all articles and product information presented on BenchChem are intended solely for informational purposes. The products available for purchase on BenchChem are specifically designed for in-vitro studies, which are conducted outside of living organisms. In-vitro studies, derived from the Latin term "in glass," involve experiments performed in controlled laboratory settings using cells or tissues. It is important to note that these products are not categorized as medicines or drugs, and they have not received approval from the FDA for the prevention, treatment, or cure of any medical condition, ailment, or disease. We must emphasize that any form of bodily introduction of these products into humans or animals is strictly prohibited by law. It is essential to adhere to these guidelines to ensure compliance with legal and ethical standards in research and experimentation.