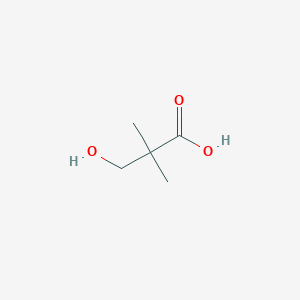
3-Hydroxy-2,2-dimethylpropanoic acid
Overview
Description
3-Hydroxy-2,2-dimethylpropanoic acid is an organic compound with the molecular formula C5H10O3 .
Synthesis Analysis
The synthesis of this compound involves several steps. For instance, the model ester was transformed into the corresponding trichloroacetimidate or acetate by the reaction with trichloroacetonitrile and acetic anhydride . N-Alkyl-3-(4-chlorophenyl)-3-hydroxy-2,2-dimethylpropanamides and methyl-2-[(3-(4-chlorophenyl)-3-hydroxy-2,2-dimethylpropanoyl)amino]alkanoates were obtained by the reaction of corresponding acid or hydrazide with amines and amino acid esters via DCC and azide coupling methods .Molecular Structure Analysis
The molecular structure of this compound can be viewed using Java or Javascript .Chemical Reactions Analysis
The chemical reactions involving this compound are complex. For instance, it can react with amines and amino acid esters via DCC and azide coupling methods to form N-Alkyl-3-(4-chlorophenyl)-3-hydroxy-2,2-dimethylpropanamides and methyl-2-[(3-(4-chlorophenyl)-3-hydroxy-2,2-dimethylpropanoyl)amino]alkanoates .Physical And Chemical Properties Analysis
This compound is soluble in water . It has a molecular weight of 118.13 .Scientific Research Applications
Cancer Therapy and Inhibitor Synthesis :
- Metal complexes derived from 3-Hydroxy-2,2-dimethylpropanoic acid show promising anti-tumor activities, specifically as inhibitors against human colorectal carcinoma cells, without inhibitory action on normal cells (Aboelmagd et al., 2021).
- Derivatives of this acid have been synthesized and tested for their antiproliferative activity against various cancer cell lines, showing potential as histone deacetylase inhibitors (HDACIs), a significant target in cancer treatment (El-Rayes et al., 2019).
- A series of compounds based on this compound selectively inhibited the proliferation of colon cancer cells, suggesting a potential role in targeted cancer therapy (Rayes et al., 2020).
Synthesis and Chemical Properties :
- The compound has been synthesized through various methods, such as hydrogen peroxide oxidation of hydroxypivaldehyde, demonstrating the versatility and accessibility of this compound in chemical synthesis (Jiang Nan-zhe, 2004).
- Research on its structure and stereochemistry, including the study of its dimer form, provides valuable insights into its chemical properties and potential applications (Santoro & Chiavarini, 1978).
- Efficient synthetic methodologies for variants of this compound have been developed, indicating its role in the synthesis of bioactive marine cyanobacterial metabolites (Nunnery et al., 2011).
Pharmaceutical Applications :
- The compound's derivatives have been studied for their potential as chelating agents for iron and aluminium, which is crucial in certain therapeutic applications (Dean et al., 2009).
- Its esters have been found to have significantly lower odor thresholds compared to their straight-chain counterparts, indicating potential applications in fragrance and flavor industries (Takeoka et al., 1995).
Future Directions
Mechanism of Action
Target of Action
It has been found that derivatives of this compound show affinity to the heat shock proteins trap1 . These proteins play a crucial role in cellular processes such as protein folding and protection against stress.
Mode of Action
Its derivatives have been shown to inhibit the proliferation of colon cancer cells . This suggests that the compound may interact with its targets, leading to changes in cellular processes that inhibit cell proliferation.
Result of Action
Its derivatives have been shown to selectively inhibit the proliferation of colon cancer cells , suggesting that it may have potential therapeutic applications in cancer treatment.
Properties
IUPAC Name |
3-hydroxy-2,2-dimethylpropanoic acid | |
---|---|---|
Source | PubChem | |
URL | https://pubchem.ncbi.nlm.nih.gov | |
Description | Data deposited in or computed by PubChem | |
InChI |
InChI=1S/C5H10O3/c1-5(2,3-6)4(7)8/h6H,3H2,1-2H3,(H,7,8) | |
Source | PubChem | |
URL | https://pubchem.ncbi.nlm.nih.gov | |
Description | Data deposited in or computed by PubChem | |
InChI Key |
RDFQSFOGKVZWKF-UHFFFAOYSA-N | |
Source | PubChem | |
URL | https://pubchem.ncbi.nlm.nih.gov | |
Description | Data deposited in or computed by PubChem | |
Canonical SMILES |
CC(C)(CO)C(=O)O | |
Source | PubChem | |
URL | https://pubchem.ncbi.nlm.nih.gov | |
Description | Data deposited in or computed by PubChem | |
Molecular Formula |
C5H10O3 | |
Source | PubChem | |
URL | https://pubchem.ncbi.nlm.nih.gov | |
Description | Data deposited in or computed by PubChem | |
DSSTOX Substance ID |
DTXSID4063616 | |
Record name | 3-Hydroxy-2,2-dimethylpropionic acid | |
Source | EPA DSSTox | |
URL | https://comptox.epa.gov/dashboard/DTXSID4063616 | |
Description | DSSTox provides a high quality public chemistry resource for supporting improved predictive toxicology. | |
Molecular Weight |
118.13 g/mol | |
Source | PubChem | |
URL | https://pubchem.ncbi.nlm.nih.gov | |
Description | Data deposited in or computed by PubChem | |
CAS No. |
4835-90-9 | |
Record name | Hydroxypivalic acid | |
Source | CAS Common Chemistry | |
URL | https://commonchemistry.cas.org/detail?cas_rn=4835-90-9 | |
Description | CAS Common Chemistry is an open community resource for accessing chemical information. Nearly 500,000 chemical substances from CAS REGISTRY cover areas of community interest, including common and frequently regulated chemicals, and those relevant to high school and undergraduate chemistry classes. This chemical information, curated by our expert scientists, is provided in alignment with our mission as a division of the American Chemical Society. | |
Explanation | The data from CAS Common Chemistry is provided under a CC-BY-NC 4.0 license, unless otherwise stated. | |
Record name | 3-Hydroxy-2,2-dimethylpropionic acid | |
Source | ChemIDplus | |
URL | https://pubchem.ncbi.nlm.nih.gov/substance/?source=chemidplus&sourceid=0004835909 | |
Description | ChemIDplus is a free, web search system that provides access to the structure and nomenclature authority files used for the identification of chemical substances cited in National Library of Medicine (NLM) databases, including the TOXNET system. | |
Record name | 4835-90-9 | |
Source | DTP/NCI | |
URL | https://dtp.cancer.gov/dtpstandard/servlet/dwindex?searchtype=NSC&outputformat=html&searchlist=115936 | |
Description | The NCI Development Therapeutics Program (DTP) provides services and resources to the academic and private-sector research communities worldwide to facilitate the discovery and development of new cancer therapeutic agents. | |
Explanation | Unless otherwise indicated, all text within NCI products is free of copyright and may be reused without our permission. Credit the National Cancer Institute as the source. | |
Record name | Propanoic acid, 3-hydroxy-2,2-dimethyl- | |
Source | EPA Chemicals under the TSCA | |
URL | https://www.epa.gov/chemicals-under-tsca | |
Description | EPA Chemicals under the Toxic Substances Control Act (TSCA) collection contains information on chemicals and their regulations under TSCA, including non-confidential content from the TSCA Chemical Substance Inventory and Chemical Data Reporting. | |
Record name | 3-Hydroxy-2,2-dimethylpropionic acid | |
Source | EPA DSSTox | |
URL | https://comptox.epa.gov/dashboard/DTXSID4063616 | |
Description | DSSTox provides a high quality public chemistry resource for supporting improved predictive toxicology. | |
Record name | 3-hydroxypivalic acid | |
Source | European Chemicals Agency (ECHA) | |
URL | https://echa.europa.eu/substance-information/-/substanceinfo/100.023.108 | |
Description | The European Chemicals Agency (ECHA) is an agency of the European Union which is the driving force among regulatory authorities in implementing the EU's groundbreaking chemicals legislation for the benefit of human health and the environment as well as for innovation and competitiveness. | |
Explanation | Use of the information, documents and data from the ECHA website is subject to the terms and conditions of this Legal Notice, and subject to other binding limitations provided for under applicable law, the information, documents and data made available on the ECHA website may be reproduced, distributed and/or used, totally or in part, for non-commercial purposes provided that ECHA is acknowledged as the source: "Source: European Chemicals Agency, http://echa.europa.eu/". Such acknowledgement must be included in each copy of the material. ECHA permits and encourages organisations and individuals to create links to the ECHA website under the following cumulative conditions: Links can only be made to webpages that provide a link to the Legal Notice page. | |
Record name | 3-HYDROXY-2,2-DIMETHYLPROPIONIC ACID | |
Source | FDA Global Substance Registration System (GSRS) | |
URL | https://gsrs.ncats.nih.gov/ginas/app/beta/substances/BJP2CXK56P | |
Description | The FDA Global Substance Registration System (GSRS) enables the efficient and accurate exchange of information on what substances are in regulated products. Instead of relying on names, which vary across regulatory domains, countries, and regions, the GSRS knowledge base makes it possible for substances to be defined by standardized, scientific descriptions. | |
Explanation | Unless otherwise noted, the contents of the FDA website (www.fda.gov), both text and graphics, are not copyrighted. They are in the public domain and may be republished, reprinted and otherwise used freely by anyone without the need to obtain permission from FDA. Credit to the U.S. Food and Drug Administration as the source is appreciated but not required. | |
Retrosynthesis Analysis
AI-Powered Synthesis Planning: Our tool employs the Template_relevance Pistachio, Template_relevance Bkms_metabolic, Template_relevance Pistachio_ringbreaker, Template_relevance Reaxys, Template_relevance Reaxys_biocatalysis model, leveraging a vast database of chemical reactions to predict feasible synthetic routes.
One-Step Synthesis Focus: Specifically designed for one-step synthesis, it provides concise and direct routes for your target compounds, streamlining the synthesis process.
Accurate Predictions: Utilizing the extensive PISTACHIO, BKMS_METABOLIC, PISTACHIO_RINGBREAKER, REAXYS, REAXYS_BIOCATALYSIS database, our tool offers high-accuracy predictions, reflecting the latest in chemical research and data.
Strategy Settings
Precursor scoring | Relevance Heuristic |
---|---|
Min. plausibility | 0.01 |
Model | Template_relevance |
Template Set | Pistachio/Bkms_metabolic/Pistachio_ringbreaker/Reaxys/Reaxys_biocatalysis |
Top-N result to add to graph | 6 |
Feasible Synthetic Routes
Disclaimer and Information on In-Vitro Research Products
Please be aware that all articles and product information presented on BenchChem are intended solely for informational purposes. The products available for purchase on BenchChem are specifically designed for in-vitro studies, which are conducted outside of living organisms. In-vitro studies, derived from the Latin term "in glass," involve experiments performed in controlled laboratory settings using cells or tissues. It is important to note that these products are not categorized as medicines or drugs, and they have not received approval from the FDA for the prevention, treatment, or cure of any medical condition, ailment, or disease. We must emphasize that any form of bodily introduction of these products into humans or animals is strictly prohibited by law. It is essential to adhere to these guidelines to ensure compliance with legal and ethical standards in research and experimentation.