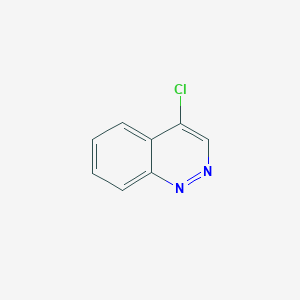
4-Chlorocinnoline
Overview
Description
4-Chlorocinnoline (C₈H₄Cl₂N₂, molecular weight: 199.034) is a bicyclic heteroaromatic compound featuring a cinnoline core (1,2-diazanaphthalene) with a chlorine substituent at the 4-position . It serves as a versatile intermediate in organic synthesis, particularly in nucleophilic substitution and cross-coupling reactions. For example, this compound reacts with amines, alkoxides, or thiols to yield derivatives such as 4-aminocinnolines, 4-alkoxycinnolines, and 4-mercaptocinnolines . Its reactivity is attributed to the electron-withdrawing effect of the chlorine atom, which activates the cinnoline ring for nucleophilic displacement.
Notably, this compound has been employed in the synthesis of bioactive compounds, including protozoan parasite growth inhibitors, via amination reactions . It also participates in palladium-catalyzed cross-coupling reactions (e.g., Suzuki and Sonogashira) to generate 4-aryl- and 4-ethynylcinnolines, which are valuable in medicinal chemistry .
Preparation Methods
Synthetic Routes and Reaction Conditions: 4-Chlorocinnoline can be synthesized through several methods. One common approach involves the reaction of cinnoline with chlorine gas in the presence of a catalyst. Another method includes the reaction of 4-chlorobenzaldehyde with hydrazine hydrate, followed by cyclization to form the cinnoline ring .
Industrial Production Methods: In industrial settings, this compound is often produced using a multi-step synthesis process. This typically involves the chlorination of cinnoline derivatives under controlled conditions to ensure high yield and purity. The reaction is usually carried out in the presence of a solvent such as dichloromethane or chloroform, with a catalyst like iron(III) chloride to facilitate the chlorination process .
Chemical Reactions Analysis
Types of Reactions: 4-Chlorocinnoline undergoes various chemical reactions, including:
Nucleophilic Substitution: The chlorine atom can be replaced by other nucleophiles, such as amines or thiols, under appropriate conditions.
Oxidation: It can be oxidized to form cinnoline N-oxides, which are useful intermediates in organic synthesis.
Common Reagents and Conditions:
Nucleophilic Substitution: Reagents like sodium hydride and primary or secondary amines in solvents such as dimethylformamide (DMF) are commonly used.
Oxidation: Oxidizing agents like hydrogen peroxide or peracids are employed.
Reduction: Reducing agents such as lithium aluminum hydride or catalytic hydrogenation are used.
Major Products:
Nucleophilic Substitution: Products include various substituted cinnolines depending on the nucleophile used.
Oxidation: Major products are cinnoline N-oxides.
Reduction: The primary product is 4-aminocinnoline.
Scientific Research Applications
Medicinal Chemistry
4-Chlorocinnoline derivatives have been investigated for their potential therapeutic effects, particularly in the treatment of diseases such as cancer and malaria.
Anticancer Activity
Research indicates that this compound exhibits cytotoxic effects against various cancer cell lines. The following table summarizes its anticancer activity:
Cell Line | IC50 (μM) | Selectivity |
---|---|---|
MCF-7 | 14.68 | High |
HCT-116 | 23.39 | Moderate |
HeLa | 50.03 | Low |
These results suggest that certain derivatives may selectively target cancer cells, offering a potential alternative to traditional chemotherapeutics.
Antimalarial Activity
The compound has also shown promising antimalarial properties, particularly against Plasmodium falciparum. The effectiveness of various derivatives is measured by their IC50 values:
Compound | IC50 (μM) | Activity Level |
---|---|---|
Compound A | 35.29 | High |
Compound B | 25.37 | High |
Compound C | 42.61 | Moderate |
Compound D | 49.68 | Moderate |
These findings indicate strong potential for developing new antimalarial agents.
Antimicrobial Activity
In addition to its anticancer and antimalarial properties, this compound has demonstrated antimicrobial activity against various bacterial strains:
Bacterial Strain | Minimum Inhibitory Concentration (MIC) μg/mL |
---|---|
Staphylococcus aureus | 12.5 |
Escherichia coli | 25 |
Pseudomonas aeruginosa | 15 |
This suggests that the compound could be valuable in developing new antimicrobial therapies.
Biological Research
The compound's unique chemical structure allows it to serve as a building block for synthesizing more complex organic molecules, making it useful in biochemical pathway studies and interactions.
Study on Antimalarial Efficacy
A study evaluated several chlorinated cinnoline derivatives, highlighting those with electron-withdrawing groups showing enhanced potency against drug-resistant strains of P. falciparum. This suggests a pathway for developing effective treatments for malaria.
Cytotoxicity Assessment
In vitro studies demonstrated that certain derivatives exhibited lower cytotoxicity compared to traditional chemotherapeutics like chloroquine, indicating a potentially safer profile for therapeutic use.
Mechanistic Insights
Research indicates that the mechanism of action may involve interference with critical metabolic pathways in parasites and cancer cells, although further studies are needed to elucidate these pathways fully.
Mechanism of Action
The mechanism of action of 4-Chlorocinnoline involves its interaction with specific molecular targets. For instance, in biological systems, it can inhibit certain enzymes by binding to their active sites, thereby blocking their activity. This inhibition can lead to various therapeutic effects, such as antimicrobial or anticancer activity . The exact pathways and molecular targets depend on the specific application and the structure of the derivatives used .
Comparison with Similar Compounds
Comparison with Structurally Related Chlorinated Heterocycles
Structural and Chemical Properties
Table 1: Key Properties of 4-Chlorocinnoline and Related Compounds
Compound | Molecular Formula | Molecular Weight | Core Structure | Chlorine Position | Key Reactivity |
---|---|---|---|---|---|
This compound | C₈H₄Cl₂N₂ | 199.034 | 1,2-Diazanaphthalene | 4 | Nucleophilic substitution, cross-coupling |
4-Chloroquinoline | C₉H₆ClN | 163.604 | Benzene fused to pyridine | 4 | Electrophilic substitution, coordination chemistry |
5-Chloroisoquinoline | C₉H₆ClN | 163.604 | Benzene fused to pyridine (iso position) | 5 | Electrophilic substitution |
2,7-Dichloro[1,8]naphthyridine | C₈H₄Cl₂N₂ | 199.034 | 1,8-Diazanaphthalene | 2,7 | Cross-coupling, metal complexation |
Key Observations:
Structural Differences: this compound and 2,7-dichloro[1,8]naphthyridine share the same molecular formula but differ in nitrogen arrangement. Cinnoline has adjacent nitrogen atoms ([1,2]-diazanaphthalene), whereas [1,8]naphthyridine has nitrogen atoms at opposite positions, leading to distinct electronic and reactivity profiles . 4-Chloroquinoline and 5-Chloroisoquinoline are monocyclic chlorinated nitrogen heterocycles with fused benzene-pyridine cores, differing in the position of the chlorine substituent.
Reactivity: this compound undergoes nucleophilic substitution (e.g., with glycidol/NaH to form epoxides ) and cross-coupling reactions (e.g., Sonogashira coupling to generate 4-ethynyl derivatives ). 4-Chloroquinoline is less reactive toward nucleophilic substitution due to the aromatic stabilization of the quinoline ring but participates in electrophilic substitutions (e.g., sulfonation, nitration) . 2,7-Dichloro[1,8]naphthyridine exhibits dual reactivity at both chlorine positions, enabling sequential functionalization .
This compound
- Amination : Reacts with amines (e.g., 3-chloro-4-((3-fluorobenzyl)oxy)aniline) under reflux to yield antiparasitic agents .
- Epoxide Formation: Reacts with glycidol/NaH to form rac-4-[(2R)-Oxiran-2-ylmethoxy]cinnoline, a precursor for aminoalcohols .
- Cross-Coupling: Suzuki coupling with boronic esters produces 4-arylcinnolines, while Sonogashira reactions generate 4-ethynyl derivatives .
4-Chloroquinoline
- Primarily used in coordination chemistry (e.g., metal-ligand complexes) and as a building block for antimalarial agents (e.g., chloroquine derivatives) .
5-Chloroisoquinoline
- Employed in the synthesis of isoquinoline alkaloids and as a ligand in catalytic systems .
Research Findings and Challenges
- Contradictions in Nomenclature: erroneously lists this compound as a synonym for 2,7-dichloro[1,8]naphthyridine, despite their distinct structures. This highlights the need for careful verification of chemical identifiers .
- Analytical Challenges: Differentiation of chlorinated heterocycles requires advanced NMR and mass spectrometry techniques, as noted in studies on cinnoline epoxides .
Biological Activity
4-Chlorocinnoline is a compound that has garnered interest in the field of medicinal chemistry due to its potential biological activities. This article explores its biological activity, including mechanisms of action, structure-activity relationships (SAR), and therapeutic applications, supported by data tables and relevant case studies.
This compound is a chlorinated derivative of cinnoline, characterized by a fused ring structure. Its chemical formula is , and it has a molecular weight of approximately 165.6 g/mol. The presence of the chlorine atom at the 4-position significantly influences its biological properties.
The biological activity of this compound is primarily attributed to its interaction with specific molecular targets, including enzymes and receptors involved in various biochemical pathways. Research indicates that it may modulate the activity of certain proteins, leading to physiological effects such as apoptosis in cancer cells and inhibition of pathogen growth.
Anticancer Properties
Recent studies have demonstrated that this compound exhibits significant anticancer properties. For instance, it has been shown to induce cytotoxic effects in various cancer cell lines, including HT-1080 fibrosarcoma cells. The compound's IC50 values indicate potent activity:
Compound | Cell Line | IC50 (µM) |
---|---|---|
This compound | HT-1080 | 1.6 |
Novel Derivative 4e | HT-1080 | 0.044 |
The derivative 4e, which incorporates modifications to the original structure, showed a marked improvement in potency (35-fold) compared to this compound itself, highlighting the importance of SAR studies in optimizing therapeutic efficacy .
Antimicrobial Activity
This compound has also been evaluated for its antimicrobial properties. It demonstrated inhibitory effects against protozoan parasites such as Leishmania major and Plasmodium falciparum. The following table summarizes the potency of related compounds:
Compound | Target Pathogen | IC50 (nM) |
---|---|---|
NEU-1017 | L. major | 68 |
NEU-924 | T. cruzi | 83 |
This compound | P. falciparum | Not specified |
These findings suggest that structural modifications can enhance the activity against specific pathogens while maintaining low toxicity profiles .
Structure-Activity Relationship (SAR)
The SAR studies conducted on derivatives of this compound reveal that substituents at various positions on the cinnoline ring significantly affect biological activity. For example:
- Chloro and Nitrile Substituents: Compounds with chlorine or nitrile at the C-5 position showed superior activity compared to those with hydrogen.
- Methoxy and Hydroxy Groups: Introduction of methoxy groups at C-3 and C-5 positions resulted in compounds with enhanced potency and selectivity against cancer cell lines .
Case Studies
-
Anticancer Efficacy:
A study evaluated the anticancer effects of several cinnoline derivatives, including this compound, demonstrating that modifications could lead to compounds with improved selectivity indices against non-cancerous cell lines (e.g., MCF-7) compared to cancerous ones. -
Antimicrobial Screening:
In another investigation focusing on antiprotozoal activity, several derivatives were screened for their ability to inhibit P. falciparum, with some exhibiting nanomolar potency against drug-resistant strains, indicating potential for therapeutic application in malaria treatment .
Q & A
Basic Research Questions
Q. What are the established synthetic routes for 4-Chlorocinnoline, and how can researchers optimize reaction yields?
- Methodological Answer : Begin with nucleophilic aromatic substitution or cross-coupling reactions, using precursors like cinnoline derivatives and chlorine sources (e.g., Cl₂, SOCl₂). Optimize parameters such as temperature (80–120°C), solvent polarity (e.g., DMF, THF), and catalyst selection (e.g., Pd/C for coupling reactions). Monitor progress via TLC or HPLC, and characterize intermediates/purified products using NMR (¹H/¹³C) and mass spectrometry . For yield optimization, employ Design of Experiments (DoE) to evaluate factorial interactions between variables .
Q. How should researchers characterize the purity and structural identity of this compound?
- Methodological Answer : Combine spectroscopic and chromatographic techniques:
- NMR : Confirm aromatic proton environments and chlorine substitution patterns.
- HPLC-MS : Assess purity (>95%) and detect trace impurities.
- Elemental Analysis : Validate empirical formula (C₉H₅ClN₂).
- X-ray Crystallography (if crystalline): Resolve 3D structure and confirm regiochemistry .
- For reproducibility, document solvent recrystallization steps and calibration standards .
Q. What preliminary biological assays are suitable for evaluating this compound’s bioactivity?
- Methodological Answer : Start with in vitro cytotoxicity assays (e.g., MTT on HeLa or HEK293 cells) and antimicrobial screening (e.g., MIC against S. aureus or E. coli). Use dose-response curves (0.1–100 µM) and include positive controls (e.g., doxorubicin for cytotoxicity). Validate results with triplicate runs and statistical tests (e.g., ANOVA with Tukey post-hoc) .
Advanced Research Questions
Q. How can researchers resolve contradictions in reported bioactivity data for this compound derivatives?
- Methodological Answer : Conduct meta-analysis of published datasets to identify variables like assay conditions (pH, incubation time) or cell line specificity. Replicate conflicting studies under standardized protocols, ensuring controlled variables (e.g., serum concentration in cell media). Use Bland-Altman plots or Cohen’s κ to quantify inter-study variability . If discrepancies persist, propose mechanistic studies (e.g., target-binding assays via SPR or ITC) to clarify bioactivity pathways .
Q. What computational strategies are effective for predicting this compound’s reactivity and binding modes?
- Methodological Answer : Apply DFT calculations (e.g., Gaussian or ORCA) to model electrophilic substitution sites and frontier molecular orbitals. For binding studies, use molecular docking (AutoDock Vina) against hypothesized targets (e.g., kinase domains) and validate with MD simulations (GROMACS) to assess stability. Cross-reference predictions with experimental SAR data .
Q. How should researchers design a mechanistic study to elucidate this compound’s role in catalytic cycles?
- Methodological Answer : Use isotopic labeling (e.g., ³⁶Cl tracer) to track chlorine transfer in catalytic reactions. Employ in situ FTIR or Raman spectroscopy to detect intermediate species. Compare kinetic data (Arrhenius plots) under varying catalyst loads and temperatures. For heterogeneous systems, conduct SEM-EDS to map surface interactions .
Q. Methodological Best Practices
- Data Integrity : Archive raw spectra/chromatograms in repositories like Zenodo, adhering to FAIR principles .
- Ethical Compliance : Obtain ethical approval for biological assays and disclose conflicts of interest per ICMJE guidelines .
- Literature Review : Use tools like SciFinder to identify knowledge gaps (e.g., limited in vivo toxicity data) and prioritize studies addressing them .
Properties
IUPAC Name |
4-chlorocinnoline | |
---|---|---|
Source | PubChem | |
URL | https://pubchem.ncbi.nlm.nih.gov | |
Description | Data deposited in or computed by PubChem | |
InChI |
InChI=1S/C8H5ClN2/c9-7-5-10-11-8-4-2-1-3-6(7)8/h1-5H | |
Source | PubChem | |
URL | https://pubchem.ncbi.nlm.nih.gov | |
Description | Data deposited in or computed by PubChem | |
InChI Key |
IJSFFUOWXQRDMS-UHFFFAOYSA-N | |
Source | PubChem | |
URL | https://pubchem.ncbi.nlm.nih.gov | |
Description | Data deposited in or computed by PubChem | |
Canonical SMILES |
C1=CC=C2C(=C1)C(=CN=N2)Cl | |
Source | PubChem | |
URL | https://pubchem.ncbi.nlm.nih.gov | |
Description | Data deposited in or computed by PubChem | |
Molecular Formula |
C8H5ClN2 | |
Source | PubChem | |
URL | https://pubchem.ncbi.nlm.nih.gov | |
Description | Data deposited in or computed by PubChem | |
DSSTOX Substance ID |
DTXSID10447987 | |
Record name | 4-chlorocinnoline | |
Source | EPA DSSTox | |
URL | https://comptox.epa.gov/dashboard/DTXSID10447987 | |
Description | DSSTox provides a high quality public chemistry resource for supporting improved predictive toxicology. | |
Molecular Weight |
164.59 g/mol | |
Source | PubChem | |
URL | https://pubchem.ncbi.nlm.nih.gov | |
Description | Data deposited in or computed by PubChem | |
CAS No. |
5152-84-1 | |
Record name | 4-Chlorocinnoline | |
Source | CAS Common Chemistry | |
URL | https://commonchemistry.cas.org/detail?cas_rn=5152-84-1 | |
Description | CAS Common Chemistry is an open community resource for accessing chemical information. Nearly 500,000 chemical substances from CAS REGISTRY cover areas of community interest, including common and frequently regulated chemicals, and those relevant to high school and undergraduate chemistry classes. This chemical information, curated by our expert scientists, is provided in alignment with our mission as a division of the American Chemical Society. | |
Explanation | The data from CAS Common Chemistry is provided under a CC-BY-NC 4.0 license, unless otherwise stated. | |
Record name | 4-chlorocinnoline | |
Source | EPA DSSTox | |
URL | https://comptox.epa.gov/dashboard/DTXSID10447987 | |
Description | DSSTox provides a high quality public chemistry resource for supporting improved predictive toxicology. | |
Record name | 4-chlorocinnoline | |
Source | European Chemicals Agency (ECHA) | |
URL | https://echa.europa.eu/information-on-chemicals | |
Description | The European Chemicals Agency (ECHA) is an agency of the European Union which is the driving force among regulatory authorities in implementing the EU's groundbreaking chemicals legislation for the benefit of human health and the environment as well as for innovation and competitiveness. | |
Explanation | Use of the information, documents and data from the ECHA website is subject to the terms and conditions of this Legal Notice, and subject to other binding limitations provided for under applicable law, the information, documents and data made available on the ECHA website may be reproduced, distributed and/or used, totally or in part, for non-commercial purposes provided that ECHA is acknowledged as the source: "Source: European Chemicals Agency, http://echa.europa.eu/". Such acknowledgement must be included in each copy of the material. ECHA permits and encourages organisations and individuals to create links to the ECHA website under the following cumulative conditions: Links can only be made to webpages that provide a link to the Legal Notice page. | |
Retrosynthesis Analysis
AI-Powered Synthesis Planning: Our tool employs the Template_relevance Pistachio, Template_relevance Bkms_metabolic, Template_relevance Pistachio_ringbreaker, Template_relevance Reaxys, Template_relevance Reaxys_biocatalysis model, leveraging a vast database of chemical reactions to predict feasible synthetic routes.
One-Step Synthesis Focus: Specifically designed for one-step synthesis, it provides concise and direct routes for your target compounds, streamlining the synthesis process.
Accurate Predictions: Utilizing the extensive PISTACHIO, BKMS_METABOLIC, PISTACHIO_RINGBREAKER, REAXYS, REAXYS_BIOCATALYSIS database, our tool offers high-accuracy predictions, reflecting the latest in chemical research and data.
Strategy Settings
Precursor scoring | Relevance Heuristic |
---|---|
Min. plausibility | 0.01 |
Model | Template_relevance |
Template Set | Pistachio/Bkms_metabolic/Pistachio_ringbreaker/Reaxys/Reaxys_biocatalysis |
Top-N result to add to graph | 6 |
Feasible Synthetic Routes
Disclaimer and Information on In-Vitro Research Products
Please be aware that all articles and product information presented on BenchChem are intended solely for informational purposes. The products available for purchase on BenchChem are specifically designed for in-vitro studies, which are conducted outside of living organisms. In-vitro studies, derived from the Latin term "in glass," involve experiments performed in controlled laboratory settings using cells or tissues. It is important to note that these products are not categorized as medicines or drugs, and they have not received approval from the FDA for the prevention, treatment, or cure of any medical condition, ailment, or disease. We must emphasize that any form of bodily introduction of these products into humans or animals is strictly prohibited by law. It is essential to adhere to these guidelines to ensure compliance with legal and ethical standards in research and experimentation.