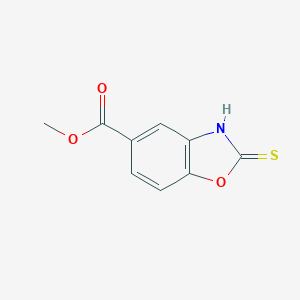
2-Mercapto-1,3-benzoxazole-5-carboxylic acid methyl ester
Overview
Description
2-Mercapto-1,3-benzoxazole-5-carboxylic acid methyl ester is a heterocyclic compound that belongs to the benzoxazole family Benzoxazoles are known for their diverse biological activities and are widely used in medicinal chemistry, agrochemicals, and material science
Mechanism of Action
Target of Action
The primary target of 2-Mercapto-1,3-benzoxazole-5-carboxylic acid methyl ester, also known as Methyl 2-Mercaptobenzo[d]oxazole-5-carboxylate, is human carbonic anhydrases (hCAs) . hCAs are zinc-containing enzymes that catalyze the reversible hydration of carbon dioxide to bicarbonate ion and proton . In humans, 12 catalytically active isoforms have been identified, which differ in tissue distribution, kinetic properties, oligomeric arrangement, and sensitivity to inhibitors .
Mode of Action
This compound inhibits hCAs by showing a peculiar binding mode . The active site of hCAs is located in a wide and deep cavity, containing a zinc ion at the bottom, tetrahedrally coordinated by three conserved His residues and a water molecule/hydroxide ion . This compound interacts with this active site, leading to the inhibition of the enzyme .
Biochemical Pathways
The inhibition of hCAs affects a wide range of physiological processes in many tissues and organs, such as pH regulation, gas exchange, ion transport, bone resorption, and fatty acid metabolism . Thus, abnormal levels or activities of these enzymes are commonly associated with various diseases .
Result of Action
The inhibition of hCAs by this compound can potentially manage a variety of disorders such as glaucoma and edema, or used as anticonvulsants, and antiobesity and antitumor drugs . .
Biochemical Analysis
Biochemical Properties
2-Mercapto-1,3-benzoxazole-5-carboxylic acid methyl ester has been shown to interact with hCAs, which are involved in a wide range of physiological processes in many tissues and organs . These enzymes catalyze the reversible hydration of carbon dioxide to bicarbonate ion and proton . The compound’s interaction with these enzymes suggests it may play a role in processes such as pH regulation, gas exchange, ion transport, bone resorption, and fatty acid metabolism .
Cellular Effects
The effects of this compound on cells are largely tied to its interaction with hCAs. By inhibiting these enzymes, it could potentially influence cell function, including impacts on cell signaling pathways, gene expression, and cellular metabolism .
Molecular Mechanism
The molecular mechanism of action of this compound involves its binding to the active site of hCAs . This binding inhibits the enzyme’s activity, which could lead to changes in gene expression and other cellular processes .
Metabolic Pathways
Given its interaction with hCAs, it may influence metabolic flux or metabolite levels .
Preparation Methods
Synthetic Routes and Reaction Conditions
The synthesis of 2-Mercapto-1,3-benzoxazole-5-carboxylic acid methyl ester typically involves the condensation of 2-aminophenol with carbon disulfide and subsequent cyclization. One common method includes the reaction of 2-aminophenol with carbon disulfide in the presence of a base such as potassium hydroxide, followed by cyclization with methyl chloroformate under reflux conditions .
Industrial Production Methods
Industrial production of this compound may involve similar synthetic routes but on a larger scale. The use of continuous flow reactors and optimized reaction conditions can enhance yield and purity. Catalysts such as metal catalysts or ionic liquids may be employed to improve reaction efficiency .
Chemical Reactions Analysis
Types of Reactions
2-Mercapto-1,3-benzoxazole-5-carboxylic acid methyl ester undergoes various chemical reactions, including:
Oxidation: The thiol group can be oxidized to form disulfides.
Reduction: The ester group can be reduced to the corresponding alcohol.
Substitution: The benzoxazole ring can undergo electrophilic substitution reactions.
Common Reagents and Conditions
Oxidation: Hydrogen peroxide or other oxidizing agents.
Reduction: Lithium aluminum hydride or sodium borohydride.
Substitution: Halogenating agents or nitrating agents under acidic conditions.
Major Products Formed
Oxidation: Disulfides.
Reduction: Alcohol derivatives.
Substitution: Halogenated or nitrated benzoxazole derivatives.
Scientific Research Applications
2-Mercapto-1,3-benzoxazole-5-carboxylic acid methyl ester has a wide range of applications in scientific research:
Chemistry: Used as a building block for the synthesis of more complex molecules.
Biology: Investigated for its potential antimicrobial and antifungal properties.
Medicine: Explored for its anticancer and anti-inflammatory activities.
Industry: Utilized in the development of new materials and as a precursor for various chemical processes.
Comparison with Similar Compounds
Similar Compounds
Benzoxazole: The parent compound with similar biological activities.
2-Mercapto-1,3-benzoxazole: Lacks the ester group but shares the thiol functionality.
5-Carboxybenzoxazole: Contains a carboxylic acid group instead of the ester.
Uniqueness
2-Mercapto-1,3-benzoxazole-5-carboxylic acid methyl ester is unique due to the presence of both the thiol and ester functionalities, which can participate in a variety of chemical reactions and interactions.
Properties
IUPAC Name |
methyl 2-sulfanylidene-3H-1,3-benzoxazole-5-carboxylate | |
---|---|---|
Source | PubChem | |
URL | https://pubchem.ncbi.nlm.nih.gov | |
Description | Data deposited in or computed by PubChem | |
InChI |
InChI=1S/C9H7NO3S/c1-12-8(11)5-2-3-7-6(4-5)10-9(14)13-7/h2-4H,1H3,(H,10,14) | |
Source | PubChem | |
URL | https://pubchem.ncbi.nlm.nih.gov | |
Description | Data deposited in or computed by PubChem | |
InChI Key |
JMJGRFGKJKXVPP-UHFFFAOYSA-N | |
Source | PubChem | |
URL | https://pubchem.ncbi.nlm.nih.gov | |
Description | Data deposited in or computed by PubChem | |
Canonical SMILES |
COC(=O)C1=CC2=C(C=C1)OC(=S)N2 | |
Source | PubChem | |
URL | https://pubchem.ncbi.nlm.nih.gov | |
Description | Data deposited in or computed by PubChem | |
Molecular Formula |
C9H7NO3S | |
Source | PubChem | |
URL | https://pubchem.ncbi.nlm.nih.gov | |
Description | Data deposited in or computed by PubChem | |
DSSTOX Substance ID |
DTXSID00415473 | |
Record name | 2-Mercapto-1,3-benzoxazole-5-carboxylic acid methyl ester | |
Source | EPA DSSTox | |
URL | https://comptox.epa.gov/dashboard/DTXSID00415473 | |
Description | DSSTox provides a high quality public chemistry resource for supporting improved predictive toxicology. | |
Molecular Weight |
209.22 g/mol | |
Source | PubChem | |
URL | https://pubchem.ncbi.nlm.nih.gov | |
Description | Data deposited in or computed by PubChem | |
CAS No. |
72730-39-3 | |
Record name | 2-Mercapto-1,3-benzoxazole-5-carboxylic acid methyl ester | |
Source | EPA DSSTox | |
URL | https://comptox.epa.gov/dashboard/DTXSID00415473 | |
Description | DSSTox provides a high quality public chemistry resource for supporting improved predictive toxicology. | |
Synthesis routes and methods I
Procedure details
Synthesis routes and methods II
Procedure details
Disclaimer and Information on In-Vitro Research Products
Please be aware that all articles and product information presented on BenchChem are intended solely for informational purposes. The products available for purchase on BenchChem are specifically designed for in-vitro studies, which are conducted outside of living organisms. In-vitro studies, derived from the Latin term "in glass," involve experiments performed in controlled laboratory settings using cells or tissues. It is important to note that these products are not categorized as medicines or drugs, and they have not received approval from the FDA for the prevention, treatment, or cure of any medical condition, ailment, or disease. We must emphasize that any form of bodily introduction of these products into humans or animals is strictly prohibited by law. It is essential to adhere to these guidelines to ensure compliance with legal and ethical standards in research and experimentation.