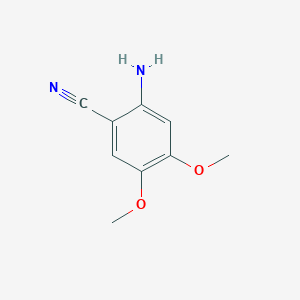
2-Amino-4,5-dimethoxybenzonitrile
Overview
Description
2-Amino-4,5-dimethoxybenzonitrile: is an organic compound with the molecular formula C9H10N2O2 . It is characterized by the presence of an amino group and two methoxy groups attached to a benzonitrile core. This compound is known for its applications in various fields, including organic synthesis and pharmaceuticals .
Preparation Methods
Synthetic Routes and Reaction Conditions:
Starting Materials: The synthesis of 2-Amino-4,5-dimethoxybenzonitrile typically begins with 4,5-dimethoxybenzonitrile.
Reaction Steps: The nitrile group is introduced through a nucleophilic substitution reaction, where an amino group is added to the aromatic ring.
Reaction Conditions: The reaction is usually carried out under controlled temperature and pressure conditions to ensure high yield and purity.
Industrial Production Methods:
Large-Scale Synthesis: Industrial production often involves the use of continuous flow reactors to maintain consistent reaction conditions.
Purification: The product is purified using techniques such as recrystallization or chromatography to achieve the desired purity levels.
Chemical Reactions Analysis
Types of Reactions:
Oxidation: 2-Amino-4,5-dimethoxybenzonitrile can undergo oxidation reactions to form corresponding quinones.
Reduction: The compound can be reduced to form amines or other reduced derivatives.
Common Reagents and Conditions:
Oxidizing Agents: Common oxidizing agents include potassium permanganate and chromium trioxide.
Reducing Agents: Sodium borohydride and lithium aluminum hydride are frequently used reducing agents.
Substitution Reagents: Halogens, alkylating agents, and acylating agents are commonly used in substitution reactions.
Major Products:
Oxidation Products: Quinones and other oxidized derivatives.
Reduction Products: Amines and other reduced compounds.
Substitution Products: Various substituted benzonitriles depending on the reagents used.
Scientific Research Applications
Chemistry:
Organic Synthesis: Used as an intermediate in the synthesis of complex organic molecules.
Catalysis: Acts as a ligand in catalytic reactions.
Biology:
Biochemical Studies: Utilized in the study of enzyme mechanisms and protein interactions.
Medicine:
Pharmaceuticals: Serves as a precursor in the synthesis of pharmaceutical compounds with potential therapeutic effects.
Industry:
Material Science: Employed in the development of advanced materials and polymers.
Mechanism of Action
Molecular Targets and Pathways:
Enzyme Inhibition: The compound can inhibit specific enzymes by binding to their active sites.
Signal Transduction: It may interfere with cellular signaling pathways, affecting various biological processes.
Comparison with Similar Compounds
- 2-Amino-3,4-dimethoxybenzonitrile
- 2-Amino-4,5-dimethoxybenzaldehyde
- 2-Amino-4,5-dimethoxybenzoic acid
Uniqueness:
- Functional Groups: The presence of both amino and methoxy groups provides unique reactivity and versatility in chemical reactions.
- Applications: Its specific structure makes it particularly useful in certain pharmaceutical and industrial applications .
Properties
IUPAC Name |
2-amino-4,5-dimethoxybenzonitrile | |
---|---|---|
Source | PubChem | |
URL | https://pubchem.ncbi.nlm.nih.gov | |
Description | Data deposited in or computed by PubChem | |
InChI |
InChI=1S/C9H10N2O2/c1-12-8-3-6(5-10)7(11)4-9(8)13-2/h3-4H,11H2,1-2H3 | |
Source | PubChem | |
URL | https://pubchem.ncbi.nlm.nih.gov | |
Description | Data deposited in or computed by PubChem | |
InChI Key |
BJAYMNUBIULRMF-UHFFFAOYSA-N | |
Source | PubChem | |
URL | https://pubchem.ncbi.nlm.nih.gov | |
Description | Data deposited in or computed by PubChem | |
Canonical SMILES |
COC1=C(C=C(C(=C1)C#N)N)OC | |
Source | PubChem | |
URL | https://pubchem.ncbi.nlm.nih.gov | |
Description | Data deposited in or computed by PubChem | |
Molecular Formula |
C9H10N2O2 | |
Source | PubChem | |
URL | https://pubchem.ncbi.nlm.nih.gov | |
Description | Data deposited in or computed by PubChem | |
DSSTOX Substance ID |
DTXSID80352892 | |
Record name | 2-Amino-4,5-dimethoxybenzonitrile | |
Source | EPA DSSTox | |
URL | https://comptox.epa.gov/dashboard/DTXSID80352892 | |
Description | DSSTox provides a high quality public chemistry resource for supporting improved predictive toxicology. | |
Molecular Weight |
178.19 g/mol | |
Source | PubChem | |
URL | https://pubchem.ncbi.nlm.nih.gov | |
Description | Data deposited in or computed by PubChem | |
CAS No. |
26961-27-3 | |
Record name | 2-Amino-4,5-dimethoxybenzonitrile | |
Source | CAS Common Chemistry | |
URL | https://commonchemistry.cas.org/detail?cas_rn=26961-27-3 | |
Description | CAS Common Chemistry is an open community resource for accessing chemical information. Nearly 500,000 chemical substances from CAS REGISTRY cover areas of community interest, including common and frequently regulated chemicals, and those relevant to high school and undergraduate chemistry classes. This chemical information, curated by our expert scientists, is provided in alignment with our mission as a division of the American Chemical Society. | |
Explanation | The data from CAS Common Chemistry is provided under a CC-BY-NC 4.0 license, unless otherwise stated. | |
Record name | 2-Amino-4,5-dimethoxybenzonitrile | |
Source | EPA DSSTox | |
URL | https://comptox.epa.gov/dashboard/DTXSID80352892 | |
Description | DSSTox provides a high quality public chemistry resource for supporting improved predictive toxicology. | |
Synthesis routes and methods
Procedure details
Retrosynthesis Analysis
AI-Powered Synthesis Planning: Our tool employs the Template_relevance Pistachio, Template_relevance Bkms_metabolic, Template_relevance Pistachio_ringbreaker, Template_relevance Reaxys, Template_relevance Reaxys_biocatalysis model, leveraging a vast database of chemical reactions to predict feasible synthetic routes.
One-Step Synthesis Focus: Specifically designed for one-step synthesis, it provides concise and direct routes for your target compounds, streamlining the synthesis process.
Accurate Predictions: Utilizing the extensive PISTACHIO, BKMS_METABOLIC, PISTACHIO_RINGBREAKER, REAXYS, REAXYS_BIOCATALYSIS database, our tool offers high-accuracy predictions, reflecting the latest in chemical research and data.
Strategy Settings
Precursor scoring | Relevance Heuristic |
---|---|
Min. plausibility | 0.01 |
Model | Template_relevance |
Template Set | Pistachio/Bkms_metabolic/Pistachio_ringbreaker/Reaxys/Reaxys_biocatalysis |
Top-N result to add to graph | 6 |
Feasible Synthetic Routes
Q1: What is the significance of the crystal structure of 2-Amino-4,5-dimethoxybenzonitrile?
A: The crystal structure of this compound reveals important information about its molecular arrangement and potential interactions. X-ray crystallography studies show that it crystallizes with two molecules in the asymmetric unit. These molecules exhibit a planar conformation and are stabilized by N-H···O hydrogen bonds in the crystal lattice. Understanding the spatial arrangement and intermolecular interactions provides insights into its physical and chemical properties, which are crucial for further applications.
Q2: How can this compound be used in organic synthesis?
A: this compound serves as a versatile building block in organic synthesis. It can undergo cyclocondensation reactions with various cyclic ketones. For example, reacting it with cyclohexanone yields 6′,7′-Dimethoxy-1′,2′-dihydrospiro[cyclohexane-1,2′-quinazolin]-4′(3′H)-one . This spiro compound, characterized by its unique structure, could have potential applications in medicinal chemistry or materials science.
Q3: Can you describe a synthetic route for Alfuzosin Hydrochloride that utilizes this compound?
A: Yes, this compound is a key starting material for synthesizing Alfuzosin Hydrochloride, a medication used to treat benign prostatic hyperplasia. The synthesis involves a five-step process:
Disclaimer and Information on In-Vitro Research Products
Please be aware that all articles and product information presented on BenchChem are intended solely for informational purposes. The products available for purchase on BenchChem are specifically designed for in-vitro studies, which are conducted outside of living organisms. In-vitro studies, derived from the Latin term "in glass," involve experiments performed in controlled laboratory settings using cells or tissues. It is important to note that these products are not categorized as medicines or drugs, and they have not received approval from the FDA for the prevention, treatment, or cure of any medical condition, ailment, or disease. We must emphasize that any form of bodily introduction of these products into humans or animals is strictly prohibited by law. It is essential to adhere to these guidelines to ensure compliance with legal and ethical standards in research and experimentation.