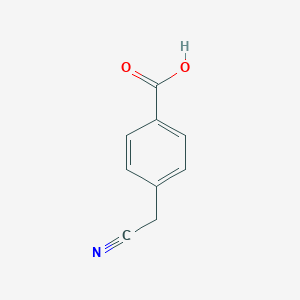
4-(Cyanomethyl)benzoic acid
Overview
Description
4-(Cyanomethyl)benzoic acid is an organic compound with the molecular formula C9H7NO2 It is characterized by a benzoic acid core substituted with a cyanomethyl group at the para position
Mechanism of Action
Target of Action
It’s known that the compound is used in dye-sensitized solar cells (dsscs), indicating its potential role in energy conversion .
Mode of Action
In the context of DSSCs, 4-(Cyanomethyl)benzoic acid-based dyes exhibit a mode of action involving electron transfer. When electron transitions from the highest occupied molecular orbital (HOMO) to the lowest unoccupied molecular orbital (LUMO), excitation results in electron transfer from the triarylamine group to the this compound moiety .
Biochemical Pathways
It’s worth noting that benzoic acids, which are the building blocks of most phenolic compounds in foods, are generally derived from corresponding cinnamic acid derivatives through the enzymatic reactions of the shikimate and phenylpropanoid pathway .
Result of Action
The result of the action of this compound is primarily observed in the context of DSSCs. A solar cell based on the this compound-based dyes exhibits good photovoltaic performance .
Preparation Methods
Synthetic Routes and Reaction Conditions: 4-(Cyanomethyl)benzoic acid can be synthesized through several methods. One common approach involves the cyanomethylation of benzoic acid derivatives. This process typically includes the reaction of benzoic acid esters with cyanomethylating agents under controlled conditions. For instance, the reaction of benzoic acid esters with trichloromethyl compounds followed by treatment with hydrogen cyanide can yield the desired product .
Industrial Production Methods: In industrial settings, the production of this compound often involves large-scale reactions using optimized conditions to ensure high yield and purity. The process may include steps such as refluxing with specific catalysts and subsequent purification through recrystallization or distillation .
Chemical Reactions Analysis
Types of Reactions: 4-(Cyanomethyl)benzoic acid undergoes various chemical reactions, including:
Oxidation: The compound can be oxidized to form corresponding carboxylic acids or other oxidized derivatives.
Reduction: Reduction reactions can convert the cyanomethyl group to an amine or other reduced forms.
Common Reagents and Conditions:
Oxidation: Common oxidizing agents include potassium permanganate and chromium trioxide.
Reduction: Reducing agents such as lithium aluminum hydride or hydrogen gas in the presence of a catalyst are often used.
Substitution: Nucleophiles like amines or alcohols can be used under basic or acidic conditions to achieve substitution reactions.
Major Products Formed:
Oxidation: Formation of carboxylic acids or aldehydes.
Reduction: Formation of amines or other reduced derivatives.
Substitution: Formation of various substituted benzoic acid derivatives.
Scientific Research Applications
4-(Cyanomethyl)benzoic acid has a wide range of applications in scientific research:
Chemistry: It is used as an intermediate in the synthesis of more complex organic molecules.
Biology: The compound can be used in the study of enzyme interactions and metabolic pathways.
Industry: The compound is used in the production of dyes, pigments, and other industrial chemicals.
Comparison with Similar Compounds
- 4-Cyanobenzoic acid
- Methyl 4-cyanobenzoate
- Ethyl 4-cyanobenzoate
- 4-Bromo-2-(cyanomethyl)benzoic acid
Comparison: 4-(Cyanomethyl)benzoic acid is unique due to the presence of the cyanomethyl group, which imparts distinct chemical properties compared to other similar compounds. For instance, 4-cyanobenzoic acid lacks the cyanomethyl group, resulting in different reactivity and applications. Methyl and ethyl 4-cyanobenzoates are esters and have different physical and chemical properties, such as solubility and boiling points .
Properties
IUPAC Name |
4-(cyanomethyl)benzoic acid | |
---|---|---|
Source | PubChem | |
URL | https://pubchem.ncbi.nlm.nih.gov | |
Description | Data deposited in or computed by PubChem | |
InChI |
InChI=1S/C9H7NO2/c10-6-5-7-1-3-8(4-2-7)9(11)12/h1-4H,5H2,(H,11,12) | |
Source | PubChem | |
URL | https://pubchem.ncbi.nlm.nih.gov | |
Description | Data deposited in or computed by PubChem | |
InChI Key |
RSGBXCFAYHOKQZ-UHFFFAOYSA-N | |
Source | PubChem | |
URL | https://pubchem.ncbi.nlm.nih.gov | |
Description | Data deposited in or computed by PubChem | |
Canonical SMILES |
C1=CC(=CC=C1CC#N)C(=O)O | |
Source | PubChem | |
URL | https://pubchem.ncbi.nlm.nih.gov | |
Description | Data deposited in or computed by PubChem | |
Molecular Formula |
C9H7NO2 | |
Source | PubChem | |
URL | https://pubchem.ncbi.nlm.nih.gov | |
Description | Data deposited in or computed by PubChem | |
DSSTOX Substance ID |
DTXSID60297095 | |
Record name | 4-(cyanomethyl)benzoic acid | |
Source | EPA DSSTox | |
URL | https://comptox.epa.gov/dashboard/DTXSID60297095 | |
Description | DSSTox provides a high quality public chemistry resource for supporting improved predictive toxicology. | |
Molecular Weight |
161.16 g/mol | |
Source | PubChem | |
URL | https://pubchem.ncbi.nlm.nih.gov | |
Description | Data deposited in or computed by PubChem | |
CAS No. |
50685-26-2 | |
Record name | 50685-26-2 | |
Source | DTP/NCI | |
URL | https://dtp.cancer.gov/dtpstandard/servlet/dwindex?searchtype=NSC&outputformat=html&searchlist=113990 | |
Description | The NCI Development Therapeutics Program (DTP) provides services and resources to the academic and private-sector research communities worldwide to facilitate the discovery and development of new cancer therapeutic agents. | |
Explanation | Unless otherwise indicated, all text within NCI products is free of copyright and may be reused without our permission. Credit the National Cancer Institute as the source. | |
Record name | 4-(cyanomethyl)benzoic acid | |
Source | EPA DSSTox | |
URL | https://comptox.epa.gov/dashboard/DTXSID60297095 | |
Description | DSSTox provides a high quality public chemistry resource for supporting improved predictive toxicology. | |
Record name | 4-(cyanomethyl)benzoic acid | |
Source | European Chemicals Agency (ECHA) | |
URL | https://echa.europa.eu/information-on-chemicals | |
Description | The European Chemicals Agency (ECHA) is an agency of the European Union which is the driving force among regulatory authorities in implementing the EU's groundbreaking chemicals legislation for the benefit of human health and the environment as well as for innovation and competitiveness. | |
Explanation | Use of the information, documents and data from the ECHA website is subject to the terms and conditions of this Legal Notice, and subject to other binding limitations provided for under applicable law, the information, documents and data made available on the ECHA website may be reproduced, distributed and/or used, totally or in part, for non-commercial purposes provided that ECHA is acknowledged as the source: "Source: European Chemicals Agency, http://echa.europa.eu/". Such acknowledgement must be included in each copy of the material. ECHA permits and encourages organisations and individuals to create links to the ECHA website under the following cumulative conditions: Links can only be made to webpages that provide a link to the Legal Notice page. | |
Synthesis routes and methods I
Procedure details
Synthesis routes and methods II
Procedure details
Synthesis routes and methods III
Procedure details
Retrosynthesis Analysis
AI-Powered Synthesis Planning: Our tool employs the Template_relevance Pistachio, Template_relevance Bkms_metabolic, Template_relevance Pistachio_ringbreaker, Template_relevance Reaxys, Template_relevance Reaxys_biocatalysis model, leveraging a vast database of chemical reactions to predict feasible synthetic routes.
One-Step Synthesis Focus: Specifically designed for one-step synthesis, it provides concise and direct routes for your target compounds, streamlining the synthesis process.
Accurate Predictions: Utilizing the extensive PISTACHIO, BKMS_METABOLIC, PISTACHIO_RINGBREAKER, REAXYS, REAXYS_BIOCATALYSIS database, our tool offers high-accuracy predictions, reflecting the latest in chemical research and data.
Strategy Settings
Precursor scoring | Relevance Heuristic |
---|---|
Min. plausibility | 0.01 |
Model | Template_relevance |
Template Set | Pistachio/Bkms_metabolic/Pistachio_ringbreaker/Reaxys/Reaxys_biocatalysis |
Top-N result to add to graph | 6 |
Feasible Synthetic Routes
Q1: What makes 4-(cyanomethyl)benzoic acid a promising component for DSSCs?
A: this compound is a promising acceptor group in organic dyes for DSSCs. Studies show its incorporation enhances the dye's light absorption properties and leads to improved photovoltaic performance compared to the more common cyanoacrylic acid acceptor []. This improvement is attributed to superior charge injection properties, which are crucial for efficient energy conversion in DSSCs [].
Q2: How does the structure of this compound-based dyes impact their performance in DSSCs?
A: Research indicates that the specific structure of dyes incorporating this compound significantly impacts their effectiveness in DSSCs. For instance, replacing a para-phenyl group with a thiophene group in conjunction with the this compound acceptor leads to enhanced solubility, broader light absorption, and improved energy conversion efficiency []. Additionally, using a dithienopyrrole unit as the central π-bridge instead of the commonly used cyclopentadithiophene linker in these dyes results in higher photovoltaic performance and longer electron lifetimes, further emphasizing the importance of structural modifications for optimizing DSSC performance [].
Q3: Have computational studies been used to investigate this compound-based dyes?
A: Yes, density functional theory (DFT) and time-dependent density functional theory (TDDFT) calculations have been used to study the electronic structure and optical properties of this compound-based dyes [, ]. These calculations help predict how the dyes will interact with light and the titanium dioxide photoanode in a DSSC, providing valuable insights for designing more efficient solar cell materials [].
Disclaimer and Information on In-Vitro Research Products
Please be aware that all articles and product information presented on BenchChem are intended solely for informational purposes. The products available for purchase on BenchChem are specifically designed for in-vitro studies, which are conducted outside of living organisms. In-vitro studies, derived from the Latin term "in glass," involve experiments performed in controlled laboratory settings using cells or tissues. It is important to note that these products are not categorized as medicines or drugs, and they have not received approval from the FDA for the prevention, treatment, or cure of any medical condition, ailment, or disease. We must emphasize that any form of bodily introduction of these products into humans or animals is strictly prohibited by law. It is essential to adhere to these guidelines to ensure compliance with legal and ethical standards in research and experimentation.