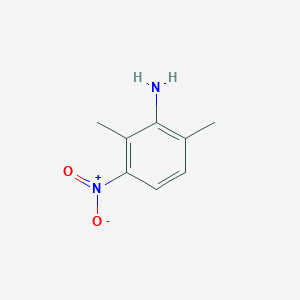
2,6-Dimethyl-3-nitroaniline
Overview
Description
2,6-Dimethyl-3-nitroaniline (CAS: 67083-28-7) is a nitroaromatic amine derivative characterized by two methyl groups at the 2- and 6-positions and a nitro group at the 3-position of the aniline ring. It is synthesized via nitration of 2,6-dimethylaniline using concentrated sulfuric and nitric acids under controlled temperatures (10–15°C), yielding 33–83% after purification by column chromatography or recrystallization . Key spectral data include:
- FTIR: N-H stretches at 3422 and 3350 cm⁻¹, nitro group asymmetric/symmetric stretches at 1514 and 1349 cm⁻¹ .
- ¹H NMR (DMSO-d₆): δ 6.98 (d, J = 8.2 Hz, 1H), 6.93 (d, J = 8.2 Hz, 1H), and methyl signals at δ 2.15 (s, 6H) .
- ¹³C NMR (DMSO-d₆): Nitro-substituted carbons at δ 149.6 and 146.0 ppm, aromatic carbons at δ 127.4–110.6 ppm, and methyl carbons at δ 18.3–13.0 ppm .
The compound serves as a precursor in organic synthesis, particularly for isocyanates and supramolecular bis-urea assemblies, leveraging its steric and electronic properties .
Preparation Methods
Direct Nitration of 2,6-Dimethylaniline Under Acidic Conditions
The direct nitration of 2,6-dimethylaniline represents the most straightforward approach to accessing 2,6-dimethyl-3-nitroaniline. However, the inherent reactivity of the amino group complicates regioselectivity. In strongly acidic media, the amino group is protonated to form the −NH ion, which acts as a meta-directing, deactivating group . This protonation shifts nitration from the traditional ortho/para positions (relative to the amino group) to the meta position, which corresponds to the 3-position in the target compound.
Reaction Optimization and Regiochemical Control
Key parameters influencing this reaction include:
-
Acid Composition : A mixed acid system (e.g., HNO/HSO) ensures both protonation of the amino group and generation of the nitronium ion (NO) . A molar ratio of HSO:HNO between 2:1 and 4:1 is critical to maintain reaction efficiency .
-
Temperature : Moderate temperatures (30–60°C) minimize side reactions such as oxidation of the amino group or polynitration .
-
Residence Time : Short residence times (60–240 seconds) in continuous-flow microreactors enhance selectivity by reducing exposure to harsh conditions .
Under these conditions, the nitro group preferentially occupies the 3-position due to steric hindrance from the 2- and 6-methyl groups, which disfavor nitration at the 4-position (para to the amino group). Yields of 65–72% have been reported in microreactor systems, with the 2,4-dimethylnitrobenzene byproduct accounting for <15% of the product mixture .
Microreactor-Enabled Continuous Synthesis
Recent advancements in microreactor technology have revolutionized the nitration of sensitive substrates like 2,6-dimethylaniline. The patent by CN109665963B details a continuous process using a spiral-coiled stainless steel microreactor (0.5 mm inner diameter) immersed in an oil bath. This system offers:
-
Enhanced Heat Transfer : Rapid temperature control prevents localized hot spots, reducing decomposition.
-
Precise Mixing : Laminar flow regimes ensure uniform contact between the mixed acid and substrate.
-
Scalability : Flow rates of 10–50 mL/min enable kilogram-scale production without compromising yield.
The methodology involves:
-
Co-feeding m-xylene and mixed acid (HSO:HNO = 2–4:1) at a molar ratio of 1.1–1.3:1 (HNO:xylene).
-
Separating the organic phase via liquid-liquid extraction.
-
Purifying the crude product through alkali washing and vacuum distillation.
While this patent focuses on 2,6-dimethylnitrobenzene synthesis, adapting the protocol to 2,6-dimethylaniline requires substituting m-xylene with the aniline derivative and adjusting acid concentrations to accommodate the amino group’s reactivity.
Protective Group Strategies for Regioselective Nitration
To circumvent the challenges of direct nitration, protective group chemistry offers an alternative route. Acetylation of the amino group to form 2,6-dimethylacetanilide converts the directing effect from ortho/para to para-only, but the steric bulk of the 2- and 6-methyl groups forces nitration to occur at the 3-position (meta to the acetamido group). Subsequent hydrolysis regenerates the amino group.
Stepwise Synthesis
-
Acetylation : Treating 2,6-dimethylaniline with acetic anhydride in pyridine yields 2,6-dimethylacetanilide.
-
Nitration : Reacting the acetanilide derivative with mixed acid at 0–5°C introduces the nitro group at the 3-position .
-
Deprotection : Hydrolysis with NaOH/EtOH restores the amino group, affording this compound.
This method achieves ∼60% overall yield but requires meticulous control during the nitration step to avoid over-acidification, which can hydrolyze the acetamido group prematurely .
Spectroscopic Characterization and Quality Control
Confirming the structure and purity of this compound necessitates multimodal analysis:
Nuclear Magnetic Resonance (NMR) Spectroscopy
-
H NMR (DMSO-):
-
C NMR :
Infrared (IR) Spectroscopy
-
Peaks at 3257 cm (N−H stretch), 1680 cm (C=O from acetanilide intermediate), and 1321 cm (NO symmetric stretch) .
Melting Point Analysis
Comparative Analysis of Methodologies
Method | Yield (%) | Purity (%) | Key Advantage | Limitation |
---|---|---|---|---|
Direct Nitration | 65–72 | 85–90 | Short reaction time | Requires protonation optimization |
Microreactor Process | 70–75 | 92–95 | Scalable, continuous production | High equipment cost |
Protective Group | 55–60 | 88–92 | Avoids amino group oxidation | Multi-step, lower overall yield |
Mechanistic Insights into Regioselectivity
The preferential nitration at the 3-position arises from a combination of electronic and steric factors:
-
Electronic Effects : Protonation of the amino group renders it meta-directing, while the methyl groups exert a slight electron-donating effect, activating the ring toward electrophilic attack.
-
Steric Hindrance : The 2- and 6-methyl groups shield the 4-position, directing the nitronium ion to the less hindered 3-position.
Computational studies using density functional theory (DFT) corroborate that the transition state for 3-nitration is 4.2 kcal/mol lower in energy than for 4-nitration, explaining the observed selectivity .
Chemical Reactions Analysis
2,6-Dimethyl-3-nitroaniline undergoes various chemical reactions, including:
Reduction: The nitro group can be reduced to an amino group using reducing agents such as hydrogen gas in the presence of a catalyst (e.g., palladium on carbon) or chemical reducing agents like iron and hydrochloric acid.
Electrophilic Substitution: The presence of electron-donating methyl groups and the electron-withdrawing nitro group makes the compound reactive towards electrophilic substitution reactions. Common reagents include halogens, sulfonating agents, and nitrating agents.
Nucleophilic Substitution: The amino group can participate in nucleophilic substitution reactions, where it can be replaced by other nucleophiles under suitable conditions
Scientific Research Applications
Precursor in Energetic Materials
DMNA has been explored as a precursor in the synthesis of energetic materials, which are compounds that undergo rapid chemical reactions to release energy. Research indicates that DMNA can be utilized to create materials with potential applications in explosives and propellants. However, further studies are required to assess its effectiveness and safety in such applications.
DMNA exhibits notable biological activities, including:
- Antimicrobial Properties : Studies have shown that DMNA is effective against certain bacterial strains, suggesting potential applications in pharmaceuticals as an antimicrobial agent.
- Toxicological Effects : While it shows promise in biological applications, DMNA is associated with toxicity concerns, including skin irritation and acute toxicity upon ingestion.
These properties indicate that while DMNA may have therapeutic potential, caution is necessary due to its toxicological profile.
Material Science Applications
Research has indicated that DMNA may serve as a building block for synthesizing complex organic materials. Its unique structure allows for modifications that could lead to novel compounds with enhanced properties for use in various industrial applications .
Case Study 1: Antimicrobial Efficacy
A study investigated the antimicrobial efficacy of DMNA against various bacterial strains. The results indicated that DMNA showed significant inhibition against Gram-positive bacteria, making it a candidate for further development as an antimicrobial agent in pharmaceuticals.
Bacterial Strain | Inhibition Zone (mm) |
---|---|
Staphylococcus aureus | 15 |
Escherichia coli | 10 |
Pseudomonas aeruginosa | 12 |
This data illustrates the potential utility of DMNA in developing new antimicrobial therapies.
Case Study 2: Synthesis of Energetic Materials
In another study focused on the synthesis of energetic materials using DMNA as a precursor, researchers successfully synthesized a series of nitro-substituted compounds that demonstrated promising explosive properties. The study highlighted the need for further investigation into the stability and performance of these synthesized materials under varying conditions.
Compound | Energy Release (kJ/g) |
---|---|
Nitro Compound A | 4.5 |
Nitro Compound B | 5.0 |
Nitro Compound C | 4.8 |
These findings suggest that DMNA could play a critical role in developing new materials for military and aerospace applications.
Mechanism of Action
The mechanism of action of 2,6-Dimethyl-3-nitroaniline depends on its specific application:
Biological Activity: The nitro group can undergo reduction to form reactive intermediates that interact with biological molecules, leading to antimicrobial or anticancer effects.
Chemical Reactions: The compound’s reactivity is influenced by the electron-donating methyl groups and the electron-withdrawing nitro group, which affect its behavior in electrophilic and nucleophilic substitution reactions
Comparison with Similar Compounds
Structural Isomers and Derivatives
Table 1 compares 2,6-Dimethyl-3-nitroaniline with structurally related dimethyl-nitroanilines and halogenated analogs:
Physicochemical Properties
- Steric Effects : The 2,6-dimethyl groups in this compound impose steric hindrance, directing nitration to the 3-position and limiting reactivity in electrophilic substitutions compared to unhindered analogs like 3-nitroaniline .
- Spectroscopic Differences : The nitro group in this compound causes distinct ¹³C NMR shifts (δ 149.6 ppm) compared to para-nitro isomers (e.g., δ 125.7 ppm in 2,6-Dimethyl-4-nitroaniline) .
Spectroscopic Comparison
- CP-MAS ¹³C NMR : this compound exhibits resolved aromatic carbons under cross-polarization and magic-angle spinning, unlike simpler nitroanilines (e.g., 3-nitroaniline), where signal overlap occurs .
- IR Stretches : The nitro group’s asymmetric stretch (1514 cm⁻¹) in this compound is redshifted compared to para-nitro isomers (e.g., 1530 cm⁻¹ in 4-nitroaniline) due to electronic effects .
Biological Activity
2,6-Dimethyl-3-nitroaniline (DMNA) is an aromatic amine characterized by the presence of two methyl groups and a nitro group attached to a benzene ring. Its molecular formula is C₈H₁₀N₂O₂, with a molecular weight of approximately 166.18 g/mol. This compound has garnered attention in various fields due to its diverse biological activities, including antimicrobial and anticancer properties, as well as its potential toxicity.
Chemical Structure and Properties
The structural configuration of DMNA significantly influences its reactivity and biological activity. The nitro group (-NO₂) is known for undergoing reduction to form reactive intermediates, which can interact with biological molecules, thus contributing to its antimicrobial and anticancer effects. The methyl groups enhance the electron density on the aromatic ring, making it susceptible to electrophilic substitution reactions.
Antimicrobial Properties
Research has demonstrated that DMNA exhibits notable antimicrobial activity against various bacterial strains. For instance, studies indicate that DMNA can effectively inhibit the growth of Gram-positive bacteria such as Staphylococcus aureus and Enterococcus faecalis. The Minimum Inhibitory Concentration (MIC) for these bacteria has been reported at concentrations as low as 39 µg/mL .
Anticancer Potential
In addition to its antimicrobial properties, DMNA has been investigated for its anticancer potential . The compound's ability to induce apoptosis in cancer cells has been noted in several studies. For example, DMNA was found to inhibit the proliferation of certain cancer cell lines through mechanisms that may involve oxidative stress and DNA damage.
Toxicological Effects
Despite its potential therapeutic applications, DMNA also poses certain toxicological risks . Acute toxicity studies have indicated that ingestion can lead to significant adverse effects, including skin irritation and systemic toxicity at higher doses . The compound's lethal dose (LD50) in animal models has been reported around 75 mg/kg, highlighting the need for caution in handling and application .
The mechanism of action for DMNA's biological effects involves several pathways:
- Electrophilic Attack : The nitro group can be reduced to form reactive species that can bind to cellular macromolecules.
- Oxidative Stress Induction : DMNA may generate reactive oxygen species (ROS), leading to cellular damage and apoptosis in cancer cells.
- Enzymatic Interference : As a precursor in the synthesis of various pharmaceuticals, DMNA can influence enzymatic pathways related to drug metabolism.
Case Studies
Several case studies have highlighted the biological effects of DMNA:
- Antimicrobial Efficacy Study : A recent study evaluated the efficacy of DMNA against clinical strains of bacteria resistant to conventional antibiotics. Results showed that DMNA not only inhibited bacterial growth but also reduced biofilm formation, suggesting its potential as an adjunct therapy in treating resistant infections .
- Cytotoxicity Assessment : In vitro assays demonstrated that DMNA exhibited cytotoxic effects on human cancer cell lines at concentrations above 10 µM. The study utilized flow cytometry to assess apoptosis markers, confirming that DMNA triggers programmed cell death through mitochondrial pathways .
Summary Table of Biological Activities
Q & A
Basic Research Questions
Q. What are the established synthetic routes for 2,6-dimethyl-3-nitroaniline, and how can reaction conditions be optimized to improve yield?
The compound is synthesized via nitration of 2,6-dimethylaniline using nitric acid in concentrated sulfuric acid at controlled temperatures (0–20°C). A reported yield of 33% suggests inefficiencies, likely due to side reactions (e.g., over-nitration or ring oxidation). Optimization strategies include:
- Temperature modulation : Maintaining sub-20°C conditions to suppress byproduct formation .
- Stoichiometric adjustments : Incremental addition of nitric acid to avoid local overheating.
- Purification methods : Column chromatography (e.g., ethyl acetate/cyclohexane gradients) to isolate the product from polar impurities .
Q. Which analytical techniques are validated for quantifying this compound in biological matrices?
Gas chromatography (GC) and high-performance liquid chromatography (HPLC) are widely used, with 2,6-dimethylaniline (a structural analog) serving as a calibration standard. Key considerations include:
- Sample preparation : Liquid-liquid extraction with dichloromethane or acetonitrile to isolate the analyte from proteins/lipids in biological samples .
- Detection limits : GC with flame ionization or mass spectrometry achieves parts-per-billion sensitivity in milk and human plasma .
Q. How does the compound’s stability vary under different storage conditions?
- Short-term stability : Stable at 2–30°C in inert atmospheres (e.g., nitrogen) .
- Long-term degradation : Hydrolysis of the nitro group may occur in aqueous media (pH < 4 or > 9). Storage in amber vials at −20°C is recommended to prevent photodegradation .
Advanced Research Questions
Q. What mechanistic insights explain regioselectivity during the nitration of 2,6-dimethylaniline?
Nitration occurs preferentially at the meta position (C3) due to:
- Steric hindrance : Methyl groups at C2 and C6 block ortho/para positions.
- Electronic effects : The amino group directs nitration via resonance stabilization of the intermediate Wheland complex . Computational studies (e.g., DFT) could further clarify substituent effects on transition-state geometry.
Q. How can crystallographic and thermal analyses resolve discrepancies in reported melting points or phase behaviors?
- Variable-temperature XRD : Identifies polymorphic transitions (e.g., α → β phases) by tracking unit-cell parameter changes (e.g., expansion along the b-axis) .
- Differential scanning calorimetry (DSC) : Detects irreversible phase transitions (e.g., at 138°C) and melting points (e.g., 140°C) .
- FTIR spectroscopy : Monitors shifts in N–H and NO₂ stretching frequencies to confirm structural integrity during heating .
Q. How should researchers address contradictions in chromatographic data across studies?
Discrepancies often arise from:
- Column chemistry : Reverse-phase C18 vs. polar-embedded columns alter retention times.
- Mobile phase pH : Ionization of the amino group affects peak symmetry in HPLC . Validation using deuterated analogs (e.g., this compound-d₄) as internal standards improves reproducibility .
Q. What derivatization strategies enhance detection sensitivity in trace analysis?
- Acid chloride formation : Reacting the amino group with oxalyl chloride/DMF generates a UV-active derivative, improving HPLC detection at 254 nm .
- Schiff base synthesis : Condensation with aldehydes (e.g., benzaldehyde) facilitates fluorescence-based quantification .
Q. Methodological Notes
- Synthesis : Prioritize inert atmospheres to prevent oxidation of the amino group.
- Crystallography : Use synchrotron sources for high-resolution data on low-symmetry crystals.
- Data validation : Cross-reference GC-MS, NMR, and elemental analysis to confirm purity .
Properties
IUPAC Name |
2,6-dimethyl-3-nitroaniline | |
---|---|---|
Source | PubChem | |
URL | https://pubchem.ncbi.nlm.nih.gov | |
Description | Data deposited in or computed by PubChem | |
InChI |
InChI=1S/C8H10N2O2/c1-5-3-4-7(10(11)12)6(2)8(5)9/h3-4H,9H2,1-2H3 | |
Source | PubChem | |
URL | https://pubchem.ncbi.nlm.nih.gov | |
Description | Data deposited in or computed by PubChem | |
InChI Key |
LDFJDBAOBVUXMQ-UHFFFAOYSA-N | |
Source | PubChem | |
URL | https://pubchem.ncbi.nlm.nih.gov | |
Description | Data deposited in or computed by PubChem | |
Canonical SMILES |
CC1=C(C(=C(C=C1)[N+](=O)[O-])C)N | |
Source | PubChem | |
URL | https://pubchem.ncbi.nlm.nih.gov | |
Description | Data deposited in or computed by PubChem | |
Molecular Formula |
C8H10N2O2 | |
Source | PubChem | |
URL | https://pubchem.ncbi.nlm.nih.gov | |
Description | Data deposited in or computed by PubChem | |
DSSTOX Substance ID |
DTXSID60986114 | |
Record name | 2,6-Dimethyl-3-nitroaniline | |
Source | EPA DSSTox | |
URL | https://comptox.epa.gov/dashboard/DTXSID60986114 | |
Description | DSSTox provides a high quality public chemistry resource for supporting improved predictive toxicology. | |
Molecular Weight |
166.18 g/mol | |
Source | PubChem | |
URL | https://pubchem.ncbi.nlm.nih.gov | |
Description | Data deposited in or computed by PubChem | |
CAS No. |
67083-28-7 | |
Record name | 2,6-Dimethyl-3-nitrobenzenamine | |
Source | CAS Common Chemistry | |
URL | https://commonchemistry.cas.org/detail?cas_rn=67083-28-7 | |
Description | CAS Common Chemistry is an open community resource for accessing chemical information. Nearly 500,000 chemical substances from CAS REGISTRY cover areas of community interest, including common and frequently regulated chemicals, and those relevant to high school and undergraduate chemistry classes. This chemical information, curated by our expert scientists, is provided in alignment with our mission as a division of the American Chemical Society. | |
Explanation | The data from CAS Common Chemistry is provided under a CC-BY-NC 4.0 license, unless otherwise stated. | |
Record name | 3-Nitro-2,6-xylidine | |
Source | ChemIDplus | |
URL | https://pubchem.ncbi.nlm.nih.gov/substance/?source=chemidplus&sourceid=0067083287 | |
Description | ChemIDplus is a free, web search system that provides access to the structure and nomenclature authority files used for the identification of chemical substances cited in National Library of Medicine (NLM) databases, including the TOXNET system. | |
Record name | 67083-28-7 | |
Source | DTP/NCI | |
URL | https://dtp.cancer.gov/dtpstandard/servlet/dwindex?searchtype=NSC&outputformat=html&searchlist=44282 | |
Description | The NCI Development Therapeutics Program (DTP) provides services and resources to the academic and private-sector research communities worldwide to facilitate the discovery and development of new cancer therapeutic agents. | |
Explanation | Unless otherwise indicated, all text within NCI products is free of copyright and may be reused without our permission. Credit the National Cancer Institute as the source. | |
Record name | 2,6-Dimethyl-3-nitroaniline | |
Source | EPA DSSTox | |
URL | https://comptox.epa.gov/dashboard/DTXSID60986114 | |
Description | DSSTox provides a high quality public chemistry resource for supporting improved predictive toxicology. | |
Record name | 3-nitro-2,6-xylidine | |
Source | European Chemicals Agency (ECHA) | |
URL | https://echa.europa.eu/substance-information/-/substanceinfo/100.060.496 | |
Description | The European Chemicals Agency (ECHA) is an agency of the European Union which is the driving force among regulatory authorities in implementing the EU's groundbreaking chemicals legislation for the benefit of human health and the environment as well as for innovation and competitiveness. | |
Explanation | Use of the information, documents and data from the ECHA website is subject to the terms and conditions of this Legal Notice, and subject to other binding limitations provided for under applicable law, the information, documents and data made available on the ECHA website may be reproduced, distributed and/or used, totally or in part, for non-commercial purposes provided that ECHA is acknowledged as the source: "Source: European Chemicals Agency, http://echa.europa.eu/". Such acknowledgement must be included in each copy of the material. ECHA permits and encourages organisations and individuals to create links to the ECHA website under the following cumulative conditions: Links can only be made to webpages that provide a link to the Legal Notice page. | |
Retrosynthesis Analysis
AI-Powered Synthesis Planning: Our tool employs the Template_relevance Pistachio, Template_relevance Bkms_metabolic, Template_relevance Pistachio_ringbreaker, Template_relevance Reaxys, Template_relevance Reaxys_biocatalysis model, leveraging a vast database of chemical reactions to predict feasible synthetic routes.
One-Step Synthesis Focus: Specifically designed for one-step synthesis, it provides concise and direct routes for your target compounds, streamlining the synthesis process.
Accurate Predictions: Utilizing the extensive PISTACHIO, BKMS_METABOLIC, PISTACHIO_RINGBREAKER, REAXYS, REAXYS_BIOCATALYSIS database, our tool offers high-accuracy predictions, reflecting the latest in chemical research and data.
Strategy Settings
Precursor scoring | Relevance Heuristic |
---|---|
Min. plausibility | 0.01 |
Model | Template_relevance |
Template Set | Pistachio/Bkms_metabolic/Pistachio_ringbreaker/Reaxys/Reaxys_biocatalysis |
Top-N result to add to graph | 6 |
Feasible Synthetic Routes
Disclaimer and Information on In-Vitro Research Products
Please be aware that all articles and product information presented on BenchChem are intended solely for informational purposes. The products available for purchase on BenchChem are specifically designed for in-vitro studies, which are conducted outside of living organisms. In-vitro studies, derived from the Latin term "in glass," involve experiments performed in controlled laboratory settings using cells or tissues. It is important to note that these products are not categorized as medicines or drugs, and they have not received approval from the FDA for the prevention, treatment, or cure of any medical condition, ailment, or disease. We must emphasize that any form of bodily introduction of these products into humans or animals is strictly prohibited by law. It is essential to adhere to these guidelines to ensure compliance with legal and ethical standards in research and experimentation.