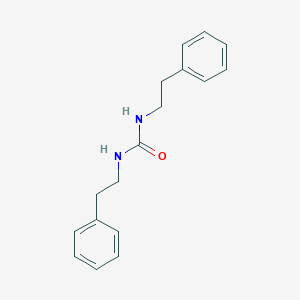
1,3-Diphenethylurea
Overview
Description
Potassium acetate, also known as potassium ethanoate, is the potassium salt of acetic acid with the chemical formula CH₃COOK. It is a hygroscopic solid at room temperature and appears as a white crystalline powder. This compound is widely used in various industries due to its versatile properties .
Preparation Methods
Synthetic Routes and Reaction Conditions: Potassium acetate can be synthesized through an acid-base neutralization reaction. This involves treating a potassium-containing base such as potassium hydroxide (KOH) or potassium carbonate (K₂CO₃) with acetic acid (CH₃COOH). The reaction is as follows: [ \text{CH}_3\text{COOH} + \text{KOH} \rightarrow \text{CH}_3\text{COOK} + \text{H}_2\text{O} ] [ 2\text{CH}_3\text{COOH} + \text{K}_2\text{CO}_3 \rightarrow 2\text{CH}_3\text{COOK} + \text{H}_2\text{O} + \text{CO}_2 ]
Industrial Production Methods: In industrial settings, potassium acetate is produced by neutralizing acetic acid with potassium hydroxide or potassium carbonate. The reaction mixture is then treated with activated carbon, filtered, and crystallized. The crystallized product is further refined and dried to obtain high-purity potassium acetate .
Chemical Reactions Analysis
Types of Reactions: Potassium acetate undergoes various chemical reactions, including:
Neutralization: As mentioned, it is formed through the neutralization of acetic acid with potassium hydroxide or potassium carbonate.
Decomposition: At high temperatures, potassium acetate decomposes to form potassium carbonate and acetone.
Substitution: It can participate in substitution reactions where the acetate ion acts as a nucleophile.
Common Reagents and Conditions:
Oxidizing Agents: Potassium acetate can react with strong oxidizing agents, leading to the formation of potassium carbonate and other by-products.
Reducing Agents: It can be reduced under specific conditions to yield different products, depending on the reagents used.
Major Products Formed:
Potassium Carbonate: Formed during the decomposition of potassium acetate.
Acetone: Another product of the decomposition reaction.
Scientific Research Applications
Antifungal Activity
1,3-Diphenethylurea has been identified as an inhibitor of Candida albicans chitin synthase, which is crucial for fungal cell wall integrity. This specificity highlights its potential as an antifungal agent without affecting similar enzymes in other organisms.
Cancer Therapeutics
Research indicates that this compound may serve as a scaffold for developing novel anticancer drugs. Its ability to induce apoptosis in cancer cells positions it as a candidate for further investigation in cancer therapy .
Anti-inflammatory Properties
Due to its role as an sEH inhibitor, this compound may be beneficial in developing treatments for conditions characterized by chronic inflammation, such as arthritis and cardiovascular diseases .
Case Study: Anticancer Activity
A study published in PLOS ONE highlighted the cytotoxic effects of this compound on various cancer cell lines. The compound was shown to significantly inhibit cell proliferation and induce apoptosis through the modulation of key signaling pathways involved in cell survival .
Case Study: Antifungal Mechanism
In another study focusing on antifungal properties, this compound was tested against Candida albicans. The results demonstrated that the compound effectively inhibited chitin synthesis by targeting chitin synthase 1, leading to compromised fungal cell walls and increased susceptibility to antifungal treatments.
Summary of Applications
Application Area | Description | Potential Impact |
---|---|---|
Antifungal Activity | Inhibits chitin synthase in Candida albicans, weakening fungal cell walls | Development of new antifungal agents |
Cancer Therapeutics | Induces apoptosis in cancer cells by modulating signaling pathways | Potential new treatments for various cancers |
Anti-inflammatory Effects | Acts as an sEH inhibitor, increasing anti-inflammatory mediators | Treatment options for chronic inflammatory diseases |
Mechanism of Action
Potassium acetate acts primarily as an electrolyte replenisher. Potassium is the major cation inside animal cells, while sodium is the major cation outside. The balance between potassium and sodium is maintained by ion pumps in the cell membrane, creating a membrane potential. This potential allows cells to generate action potentials, which are critical for neurotransmission, muscle contraction, and heart function .
Comparison with Similar Compounds
Sodium Acetate (CH₃COONa): Similar in structure but contains sodium instead of potassium. Used in similar applications but has different solubility and reactivity properties.
Calcium Acetate (Ca(CH₃COO)₂): Used as a food additive and in the treatment of hyperphosphatemia in patients with kidney disease.
Magnesium Acetate (Mg(CH₃COO)₂): Employed in textile and leather industries and as a catalyst in chemical reactions.
Uniqueness of Potassium Acetate: Potassium acetate is preferred in applications where potassium ions are beneficial, such as in medical treatments for hypokalemia. Its lower corrosiveness compared to other deicers makes it ideal for use on airport runways .
Biological Activity
1,3-Diphenethylurea is a compound that has garnered attention for its diverse biological activities, particularly in the fields of oncology, anti-inflammatory responses, and metabolic regulation. This article explores its mechanisms of action, therapeutic potential, and relevant case studies.
Overview
This compound (CAS Number: 5467-84-5) is a urea derivative known for its ability to interact with various biomolecules. Its primary mechanism involves inhibition of specific enzymes and modulation of cellular pathways. Recent studies have highlighted its cytotoxic effects against cancer cell lines and its role in metabolic processes.
Cytotoxicity Against Cancer Cells
This compound has demonstrated effective cytotoxicity against various human cancer cell lines. It primarily targets epoxide hydrolase , an enzyme involved in the metabolism of xenobiotics and drugs, leading to altered cellular functions and apoptosis in cancer cells.
Inhibition of Chitin Synthase
This compound has been identified as an inhibitor of Candida albicans Chitin Synthase 1 (CaCHSIp) , crucial for fungal cell wall integrity. By disrupting chitin synthesis, it weakens the fungal cell wall, potentially leading to cell death while sparing similar enzymes in other organisms like Saccharomyces cerevisiae, indicating a degree of selectivity.
Antitumor Properties
Recent research indicates that derivatives of this compound exhibit significant antitumor activity. For instance, a study reported that Schiff bases derived from this compound showed strong inhibition against SARS-CoV-2 spike protein with inhibitory values ranging from 79.60% to 96.00%, suggesting potential applications in viral infections alongside their anticancer properties .
Anti-inflammatory Effects
This compound has also been suggested to possess anti-inflammatory properties. Its interaction with epoxide hydrolase may contribute to reduced inflammation by modulating the levels of bioactive lipids involved in inflammatory responses .
Metabolic Regulation
In metabolic studies, it was found that this compound promotes adipocyte differentiation in pre-adipocytes. This effect is mediated through the activation of peroxisome proliferator-activated receptor gamma (PPARγ), a key regulator in adipogenesis. The compound significantly increased the expression of adipogenic markers such as aP2 and adiponectin in treated cells .
Case Study: Antidiabetic Activity
A study focused on the synthesis of Schiff bases from this compound revealed their potential as α-glucosidase inhibitors , which are crucial for managing type 2 diabetes mellitus (T2DM). The synthesized compounds exhibited IC50 values ranging from 2.14 to 115 µM, indicating strong inhibitory activity compared to acarbose, a known α-glucosidase inhibitor .
Compound | IC50 (µM) | Activity |
---|---|---|
Compound 3a | 2.14 | Strong α-glucosidase inhibitor |
Compound 3b | 45.00 | Moderate α-glucosidase inhibitor |
Compound 3c | 115.00 | Weak α-glucosidase inhibitor |
Case Study: Adipocyte Differentiation
In another investigation involving 3T3-L1 cells, treatment with this compound led to a significant increase in adipocyte differentiation markers. The compound enhanced the expression of PPARγ and other adipogenic genes in a dose-dependent manner .
Q & A
Basic Questions
Q. What synthetic routes are commonly employed to produce 1,3-Diphenylurea for laboratory research?
- Methodological Answer: 1,3-Diphenylurea is synthesized via the reaction of aniline with phosgene (COCl₂) in toluene. The phosgene reacts stoichiometrically with two equivalents of aniline to form the stable urea derivative. This method is validated by its use in environmental monitoring, where 1,3-Diphenylurea serves as a stable derivative for phosgene detection . Researchers must ensure proper ventilation and safety protocols due to phosgene's acute toxicity.
Q. Which analytical techniques are recommended for quantifying 1,3-Diphenylurea in environmental or biological samples?
- Methodological Answer: High-performance liquid chromatography (HPLC) paired with UV-Vis detection is widely used. For example, in ambient air analysis, 1,3-Diphenylurea derivatives are separated using a C18 column with a mobile phase of acetonitrile/water (70:30 v/v) and detected at 254 nm. Calibration curves are constructed using certified reference materials to ensure accuracy . Mass spectrometry (LC-MS) is recommended for trace-level quantification in complex matrices .
Q. What safety measures are critical when handling 1,3-Diphenylurea in laboratory settings?
- Methodological Answer:
- Personal Protective Equipment (PPE): Nitrile gloves, lab coats, and safety goggles. Gloves must resist permeation (e.g., Viton® for prolonged exposure) .
- Exposure Control: Use fume hoods to avoid inhalation of dust. Avoid skin contact; if exposed, rinse with water for 15 minutes and seek medical advice .
- Storage: Store in a cool, dry place away from oxidizers and ignition sources. Conduct regular risk assessments per OSHA HCS standards .
Advanced Questions
Q. How do structural modifications to the phenyl groups in 1,3-Diphenylurea influence its reactivity and bioactivity?
- Methodological Answer: Substituent effects can be systematically studied via Hammett plots or computational modeling (e.g., DFT). For instance, electron-withdrawing groups (e.g., –Cl) on the phenyl rings increase electrophilicity, altering hydrogen-bonding interactions critical for enzyme inhibition. Comparative studies with analogs like 1-(3-Chloro-4-methylphenyl)-3-(2,4,6-trimethylphenyl)urea ( ) reveal enhanced stability and target affinity. Researchers should synthesize derivatives via Ullmann coupling or nucleophilic substitution, followed by bioactivity assays (e.g., kinase inhibition) .
Q. How can discrepancies in reported melting points or solubility data for 1,3-Diphenylurea be resolved?
- Methodological Answer: Variations often arise from impurities or polymorphic forms. To address this:
- Purification: Recrystallize from ethanol/water mixtures (80:20) and validate purity via HPLC (>99%) .
- Thermal Analysis: Use differential scanning calorimetry (DSC) to identify polymorphs. For example, the reported melting point of 234–236°C () should align with a single endothermic peak in DSC .
- Inter-lab Validation: Collaborate with certified laboratories using ASTM/NIST protocols for cross-verification .
Q. What mechanistic insights explain the stability of 1,3-Diphenylurea in environmental matrices?
- Methodological Answer: The compound’s stability under ambient conditions is attributed to its rigid planar structure and strong intermolecular hydrogen bonding. Degradation studies under UV irradiation or microbial action (e.g., soil microcosms) show negligible breakdown over 30 days. Researchers should employ accelerated stability testing (40°C/75% RH) and monitor degradation products via LC-MS/MS. Evidence from phosgene detection methods confirms its persistence as a reaction product .
Properties
IUPAC Name |
1,3-bis(2-phenylethyl)urea | |
---|---|---|
Source | PubChem | |
URL | https://pubchem.ncbi.nlm.nih.gov | |
Description | Data deposited in or computed by PubChem | |
InChI |
InChI=1S/C17H20N2O/c20-17(18-13-11-15-7-3-1-4-8-15)19-14-12-16-9-5-2-6-10-16/h1-10H,11-14H2,(H2,18,19,20) | |
Source | PubChem | |
URL | https://pubchem.ncbi.nlm.nih.gov | |
Description | Data deposited in or computed by PubChem | |
InChI Key |
VMQLWVGHPWFHEI-UHFFFAOYSA-N | |
Source | PubChem | |
URL | https://pubchem.ncbi.nlm.nih.gov | |
Description | Data deposited in or computed by PubChem | |
Canonical SMILES |
C1=CC=C(C=C1)CCNC(=O)NCCC2=CC=CC=C2 | |
Source | PubChem | |
URL | https://pubchem.ncbi.nlm.nih.gov | |
Description | Data deposited in or computed by PubChem | |
Molecular Formula |
C17H20N2O | |
Source | PubChem | |
URL | https://pubchem.ncbi.nlm.nih.gov | |
Description | Data deposited in or computed by PubChem | |
DSSTOX Substance ID |
DTXSID00203130 | |
Record name | 1,3-Diphenethylurea | |
Source | EPA DSSTox | |
URL | https://comptox.epa.gov/dashboard/DTXSID00203130 | |
Description | DSSTox provides a high quality public chemistry resource for supporting improved predictive toxicology. | |
Molecular Weight |
268.35 g/mol | |
Source | PubChem | |
URL | https://pubchem.ncbi.nlm.nih.gov | |
Description | Data deposited in or computed by PubChem | |
CAS No. |
5467-84-5 | |
Record name | N,N′-Bis(2-phenylethyl)urea | |
Source | CAS Common Chemistry | |
URL | https://commonchemistry.cas.org/detail?cas_rn=5467-84-5 | |
Description | CAS Common Chemistry is an open community resource for accessing chemical information. Nearly 500,000 chemical substances from CAS REGISTRY cover areas of community interest, including common and frequently regulated chemicals, and those relevant to high school and undergraduate chemistry classes. This chemical information, curated by our expert scientists, is provided in alignment with our mission as a division of the American Chemical Society. | |
Explanation | The data from CAS Common Chemistry is provided under a CC-BY-NC 4.0 license, unless otherwise stated. | |
Record name | 1,3-Diphenethylurea | |
Source | ChemIDplus | |
URL | https://pubchem.ncbi.nlm.nih.gov/substance/?source=chemidplus&sourceid=0005467845 | |
Description | ChemIDplus is a free, web search system that provides access to the structure and nomenclature authority files used for the identification of chemical substances cited in National Library of Medicine (NLM) databases, including the TOXNET system. | |
Record name | NSC25433 | |
Source | DTP/NCI | |
URL | https://dtp.cancer.gov/dtpstandard/servlet/dwindex?searchtype=NSC&outputformat=html&searchlist=25433 | |
Description | The NCI Development Therapeutics Program (DTP) provides services and resources to the academic and private-sector research communities worldwide to facilitate the discovery and development of new cancer therapeutic agents. | |
Explanation | Unless otherwise indicated, all text within NCI products is free of copyright and may be reused without our permission. Credit the National Cancer Institute as the source. | |
Record name | 1,3-Diphenethylurea | |
Source | EPA DSSTox | |
URL | https://comptox.epa.gov/dashboard/DTXSID00203130 | |
Description | DSSTox provides a high quality public chemistry resource for supporting improved predictive toxicology. | |
Record name | 1,3-DIPHENETHYLUREA | |
Source | FDA Global Substance Registration System (GSRS) | |
URL | https://gsrs.ncats.nih.gov/ginas/app/beta/substances/9J0Y1CP1F8 | |
Description | The FDA Global Substance Registration System (GSRS) enables the efficient and accurate exchange of information on what substances are in regulated products. Instead of relying on names, which vary across regulatory domains, countries, and regions, the GSRS knowledge base makes it possible for substances to be defined by standardized, scientific descriptions. | |
Explanation | Unless otherwise noted, the contents of the FDA website (www.fda.gov), both text and graphics, are not copyrighted. They are in the public domain and may be republished, reprinted and otherwise used freely by anyone without the need to obtain permission from FDA. Credit to the U.S. Food and Drug Administration as the source is appreciated but not required. | |
Retrosynthesis Analysis
AI-Powered Synthesis Planning: Our tool employs the Template_relevance Pistachio, Template_relevance Bkms_metabolic, Template_relevance Pistachio_ringbreaker, Template_relevance Reaxys, Template_relevance Reaxys_biocatalysis model, leveraging a vast database of chemical reactions to predict feasible synthetic routes.
One-Step Synthesis Focus: Specifically designed for one-step synthesis, it provides concise and direct routes for your target compounds, streamlining the synthesis process.
Accurate Predictions: Utilizing the extensive PISTACHIO, BKMS_METABOLIC, PISTACHIO_RINGBREAKER, REAXYS, REAXYS_BIOCATALYSIS database, our tool offers high-accuracy predictions, reflecting the latest in chemical research and data.
Strategy Settings
Precursor scoring | Relevance Heuristic |
---|---|
Min. plausibility | 0.01 |
Model | Template_relevance |
Template Set | Pistachio/Bkms_metabolic/Pistachio_ringbreaker/Reaxys/Reaxys_biocatalysis |
Top-N result to add to graph | 6 |
Feasible Synthetic Routes
Disclaimer and Information on In-Vitro Research Products
Please be aware that all articles and product information presented on BenchChem are intended solely for informational purposes. The products available for purchase on BenchChem are specifically designed for in-vitro studies, which are conducted outside of living organisms. In-vitro studies, derived from the Latin term "in glass," involve experiments performed in controlled laboratory settings using cells or tissues. It is important to note that these products are not categorized as medicines or drugs, and they have not received approval from the FDA for the prevention, treatment, or cure of any medical condition, ailment, or disease. We must emphasize that any form of bodily introduction of these products into humans or animals is strictly prohibited by law. It is essential to adhere to these guidelines to ensure compliance with legal and ethical standards in research and experimentation.