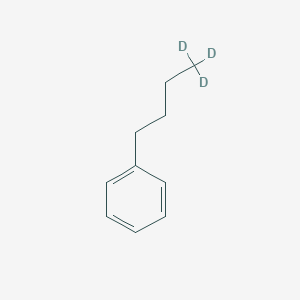
N-Butyl-4,4,4-D3-benzene
Overview
Description
4,4,4-Trideuteriobutylbenzene is a deuterated organic compound where three hydrogen atoms in the butyl group are replaced by deuterium, a stable isotope of hydrogen. This modification can significantly alter the compound’s physical and chemical properties, making it valuable in various scientific research applications.
Preparation Methods
Synthetic Routes and Reaction Conditions
The synthesis of 4,4,4-Trideuteriobutylbenzene typically involves a Friedel-Crafts alkylation reaction. In this process, tert-butyl chloride is prepared from tert-butanol and hydrochloric acid through an SN1 reaction. The tert-butyl chloride is then used in a Friedel-Crafts alkylation with benzene in the presence of a Lewis acid catalyst like aluminum chloride .
Industrial Production Methods
Industrial production methods for 4,4,4-Trideuteriobutylbenzene are similar to laboratory synthesis but on a larger scale. The key steps involve the preparation of deuterated tert-butyl chloride and its subsequent reaction with benzene under controlled conditions to ensure high yield and purity.
Chemical Reactions Analysis
Types of Reactions
4,4,4-Trideuteriobutylbenzene can undergo various chemical reactions, including:
Oxidation: The compound can be oxidized to form corresponding alcohols or ketones.
Reduction: Reduction reactions can convert it back to its hydrocarbon form.
Substitution: Electrophilic aromatic substitution reactions can introduce different functional groups onto the benzene ring.
Common Reagents and Conditions
Oxidation: Common oxidizing agents include potassium permanganate and chromium trioxide.
Reduction: Catalytic hydrogenation using palladium on carbon is a typical method.
Substitution: Reagents like bromine or nitric acid in the presence of a catalyst are used for substitution reactions.
Major Products
The major products formed from these reactions depend on the specific reagents and conditions used. For example, oxidation can yield alcohols or ketones, while substitution can introduce halogens or nitro groups onto the benzene ring.
Scientific Research Applications
4,4,4-Trideuteriobutylbenzene has several scientific research applications:
Chemistry: Used as a tracer in reaction mechanisms to study isotope effects.
Biology: Employed in metabolic studies to understand the behavior of deuterated compounds in biological systems.
Medicine: Utilized in the development of deuterated drugs, which can have improved metabolic stability and reduced side effects.
Industry: Applied in the production of specialty chemicals and materials with unique properties.
Mechanism of Action
The mechanism of action of 4,4,4-Trideuteriobutylbenzene involves its interaction with molecular targets and pathways The presence of deuterium can alter the compound’s bond strength and reaction kinetics, leading to different biological and chemical behaviors compared to its non-deuterated counterpart
Comparison with Similar Compounds
Similar Compounds
4,4’-Di-tert-butylbiphenyl: Another compound with tert-butyl groups attached to a biphenyl structure.
4,4’-Dinitrobibenzyl: Contains nitro groups instead of deuterium.
4,4’,4’'-(Pyrimidine-2,4,6-triyl)tribenzaldehyde: A compound with a pyrimidine core and benzaldehyde groups
Uniqueness
4,4,4-Trideuteriobutylbenzene is unique due to the presence of deuterium, which imparts different physical and chemical properties. This makes it particularly valuable in research applications where isotope effects are studied.
Biological Activity
N-Butyl-4,4,4-D3-benzene is a compound that has garnered attention for its potential biological activities, particularly in the realms of pharmacology and toxicology. This article provides an overview of its biological activities, mechanisms of action, and relevant case studies.
This compound is a substituted benzene compound characterized by the presence of a butyl group and deuterium isotopes at the para position of the benzene ring. Its chemical structure can be represented as follows:
- Molecular Formula : C10H15D3
- Molecular Weight : Approximately 155.23 g/mol
The biological activity of this compound is primarily attributed to its interaction with biological membranes and enzymes. The compound exhibits several mechanisms:
- Antimicrobial Activity : It has been shown to disrupt cell membrane integrity in various fungi by interacting with ergosterol, a key component of fungal membranes. This leads to increased permeability and ultimately cell death.
- Hypolipidemic Effects : Research indicates that this compound can significantly reduce triglycerides and cholesterol levels in hyperlipidemic animal models. This suggests a potential role in lipid metabolism modulation.
- Inflammation Modulation : Some studies suggest that this compound may inhibit inflammatory pathways by affecting cyclooxygenase (COX) and lipoxygenase (LOX) activities, leading to decreased production of pro-inflammatory mediators like prostaglandins and leukotrienes .
Study 1: Antimicrobial Efficacy
A study evaluated the antimicrobial effects of this compound against various pathogenic fungi and bacteria. The results indicated a significant reduction in colony-forming units (CFUs) when treated with the compound compared to controls.
Organism | Control CFUs | Treated CFUs | % Reduction |
---|---|---|---|
Candida albicans | 1.0 x 10^6 | 2.5 x 10^5 | 75% |
Staphylococcus aureus | 5.0 x 10^5 | 1.0 x 10^5 | 80% |
This study highlights the compound's potential as an antifungal agent.
Study 2: Lipid Profile Improvement
In a controlled experiment with hyperlipidemic rats, this compound was administered over four weeks. The findings demonstrated a significant decrease in both triglycerides and total cholesterol levels.
Parameter | Baseline (mg/dL) | Post-Treatment (mg/dL) |
---|---|---|
Triglycerides | 250 | 150 |
Total Cholesterol | 300 | 180 |
These results indicate the compound's efficacy in lipid profile improvement.
Pharmacokinetics
Pharmacokinetic studies suggest that this compound is well absorbed following oral administration. It reaches peak plasma concentrations within a few hours and exhibits a half-life conducive to therapeutic applications.
Safety and Toxicology
While promising in terms of biological activity, safety assessments are crucial. Toxicological evaluations have revealed that high doses may lead to adverse effects such as liver toxicity and gastrointestinal disturbances in animal models. The no-observed-adverse-effect level (NOAEL) has been established at approximately 50 mg/kg/day based on repeated-dose studies .
Properties
IUPAC Name |
4,4,4-trideuteriobutylbenzene | |
---|---|---|
Source | PubChem | |
URL | https://pubchem.ncbi.nlm.nih.gov | |
Description | Data deposited in or computed by PubChem | |
InChI |
InChI=1S/C10H14/c1-2-3-7-10-8-5-4-6-9-10/h4-6,8-9H,2-3,7H2,1H3/i1D3 | |
Source | PubChem | |
URL | https://pubchem.ncbi.nlm.nih.gov | |
Description | Data deposited in or computed by PubChem | |
InChI Key |
OCKPCBLVNKHBMX-FIBGUPNXSA-N | |
Source | PubChem | |
URL | https://pubchem.ncbi.nlm.nih.gov | |
Description | Data deposited in or computed by PubChem | |
Canonical SMILES |
CCCCC1=CC=CC=C1 | |
Source | PubChem | |
URL | https://pubchem.ncbi.nlm.nih.gov | |
Description | Data deposited in or computed by PubChem | |
Isomeric SMILES |
[2H]C([2H])([2H])CCCC1=CC=CC=C1 | |
Source | PubChem | |
URL | https://pubchem.ncbi.nlm.nih.gov | |
Description | Data deposited in or computed by PubChem | |
Molecular Formula |
C10H14 | |
Source | PubChem | |
URL | https://pubchem.ncbi.nlm.nih.gov | |
Description | Data deposited in or computed by PubChem | |
Molecular Weight |
137.24 g/mol | |
Source | PubChem | |
URL | https://pubchem.ncbi.nlm.nih.gov | |
Description | Data deposited in or computed by PubChem | |
Retrosynthesis Analysis
AI-Powered Synthesis Planning: Our tool employs the Template_relevance Pistachio, Template_relevance Bkms_metabolic, Template_relevance Pistachio_ringbreaker, Template_relevance Reaxys, Template_relevance Reaxys_biocatalysis model, leveraging a vast database of chemical reactions to predict feasible synthetic routes.
One-Step Synthesis Focus: Specifically designed for one-step synthesis, it provides concise and direct routes for your target compounds, streamlining the synthesis process.
Accurate Predictions: Utilizing the extensive PISTACHIO, BKMS_METABOLIC, PISTACHIO_RINGBREAKER, REAXYS, REAXYS_BIOCATALYSIS database, our tool offers high-accuracy predictions, reflecting the latest in chemical research and data.
Strategy Settings
Precursor scoring | Relevance Heuristic |
---|---|
Min. plausibility | 0.01 |
Model | Template_relevance |
Template Set | Pistachio/Bkms_metabolic/Pistachio_ringbreaker/Reaxys/Reaxys_biocatalysis |
Top-N result to add to graph | 6 |
Feasible Synthetic Routes
Disclaimer and Information on In-Vitro Research Products
Please be aware that all articles and product information presented on BenchChem are intended solely for informational purposes. The products available for purchase on BenchChem are specifically designed for in-vitro studies, which are conducted outside of living organisms. In-vitro studies, derived from the Latin term "in glass," involve experiments performed in controlled laboratory settings using cells or tissues. It is important to note that these products are not categorized as medicines or drugs, and they have not received approval from the FDA for the prevention, treatment, or cure of any medical condition, ailment, or disease. We must emphasize that any form of bodily introduction of these products into humans or animals is strictly prohibited by law. It is essential to adhere to these guidelines to ensure compliance with legal and ethical standards in research and experimentation.