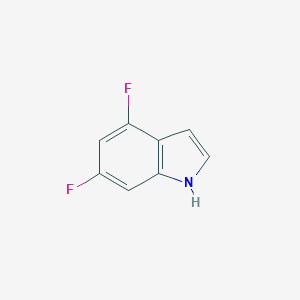
4,6-Difluoroindole
Overview
Description
4,6-Difluoroindole is a chemical compound with the molecular formula C8H5F2N . It is an important raw material and intermediate used in Organic Synthesis, Pharmaceuticals, Agrochemicals, and Dyestuffs . It is stored in a dark place, sealed in dry, at 2-8°C .
Molecular Structure Analysis
The molecular structure of this compound consists of a total of 17 bonds. There are 12 non-H bonds, 10 multiple bonds, 10 aromatic bonds, 1 five-membered ring, 1 six-membered ring, 1 nine-membered ring, and 1 Pyrrole .Physical And Chemical Properties Analysis
This compound has a molecular weight of 153.13 . It is a liquid at room temperature . It is sparingly soluble, with a solubility of 0.062 g/L at 25°C .Scientific Research Applications
Polyfluorinated Heterocyclic Compounds : T.D. Petrova et al. (1970) investigated the reactions of 4,5,6,7-tetrafluoroindole, including formylation, aminomethylation, and bromination, which proceed at the 3 position. This study contributes to the understanding of substitution reactions in polyfluorinated indoles (Petrova, Savchenko, Shchegoleva, Ardyukova, & Yakobson, 1970).
Synthesis of Difluoroindolecarboxylic Acid Ethyl Esters : R. Silvestri et al. (2004) explored synthetic approaches to ethyl 4,5-difluoroindole-2-carboxylate and ethyl 5,6-difluoroindole-2-carboxylate, providing insight into the preparation of difluoroindole derivatives (Silvestri, Artico, Regina, & Martino, 2004).
Zwitterionic and Anionic Forms of a Serotonin Analog : G. Rudnick et al. (1989) studied 4,6-Difluoroserotonin, a serotonin analog, as a substrate for biogenic amine transporters. This research provides insights into the biochemical interactions and transport mechanisms of fluoroindole derivatives (Rudnick, Kirk, Fishkes, & Schuldiner, 1989).
Safety and Hazards
Mechanism of Action
Target of Action
4,6-Difluoroindole is a promising compound in the field of medicinal chemistry. It has been shown to selectively target cancer cells, leaving healthy cells unaffected . In the context of tuberculosis treatment, it has been found to target the mycobacterial membrane protein large 3 transporter (MmpL3) .
Mode of Action
It is known that it interacts with its targets, leading to changes that inhibit the growth of targeted cells . For instance, in the case of Mycobacterium tuberculosis, it interacts with the MmpL3 transporter, disrupting its function and inhibiting the growth of the bacteria .
Biochemical Pathways
Given its interaction with the mmpl3 transporter in mycobacterium tuberculosis, it can be inferred that it affects the pathways associated with this transporter .
Pharmacokinetics
It has been suggested that it has high gastrointestinal absorption and is bbb permeant . Its lipophilicity, as indicated by its Log Po/w (iLOGP) value of 1.66, suggests that it may have good bioavailability .
Result of Action
The molecular and cellular effects of this compound’s action are primarily its inhibitory effects on the growth of targeted cells. In the context of cancer treatment, it selectively targets and inhibits the growth of cancer cells . In the treatment of tuberculosis, it inhibits the growth of Mycobacterium tuberculosis by interacting with the MmpL3 transporter .
Action Environment
It is known to be air sensitive and should be kept at room temperature
properties
IUPAC Name |
4,6-difluoro-1H-indole | |
---|---|---|
Source | PubChem | |
URL | https://pubchem.ncbi.nlm.nih.gov | |
Description | Data deposited in or computed by PubChem | |
InChI |
InChI=1S/C8H5F2N/c9-5-3-7(10)6-1-2-11-8(6)4-5/h1-4,11H | |
Source | PubChem | |
URL | https://pubchem.ncbi.nlm.nih.gov | |
Description | Data deposited in or computed by PubChem | |
InChI Key |
MHICCULQVCEWFH-UHFFFAOYSA-N | |
Source | PubChem | |
URL | https://pubchem.ncbi.nlm.nih.gov | |
Description | Data deposited in or computed by PubChem | |
Canonical SMILES |
C1=CNC2=C1C(=CC(=C2)F)F | |
Source | PubChem | |
URL | https://pubchem.ncbi.nlm.nih.gov | |
Description | Data deposited in or computed by PubChem | |
Molecular Formula |
C8H5F2N | |
Source | PubChem | |
URL | https://pubchem.ncbi.nlm.nih.gov | |
Description | Data deposited in or computed by PubChem | |
DSSTOX Substance ID |
DTXSID40381103 | |
Record name | 4,6-Difluoroindole | |
Source | EPA DSSTox | |
URL | https://comptox.epa.gov/dashboard/DTXSID40381103 | |
Description | DSSTox provides a high quality public chemistry resource for supporting improved predictive toxicology. | |
Molecular Weight |
153.13 g/mol | |
Source | PubChem | |
URL | https://pubchem.ncbi.nlm.nih.gov | |
Description | Data deposited in or computed by PubChem | |
CAS RN |
199526-97-1 | |
Record name | 4,6-Difluoroindole | |
Source | EPA DSSTox | |
URL | https://comptox.epa.gov/dashboard/DTXSID40381103 | |
Description | DSSTox provides a high quality public chemistry resource for supporting improved predictive toxicology. | |
Record name | 4,6-Difluoro-1H-indole | |
Source | European Chemicals Agency (ECHA) | |
URL | https://echa.europa.eu/information-on-chemicals | |
Description | The European Chemicals Agency (ECHA) is an agency of the European Union which is the driving force among regulatory authorities in implementing the EU's groundbreaking chemicals legislation for the benefit of human health and the environment as well as for innovation and competitiveness. | |
Explanation | Use of the information, documents and data from the ECHA website is subject to the terms and conditions of this Legal Notice, and subject to other binding limitations provided for under applicable law, the information, documents and data made available on the ECHA website may be reproduced, distributed and/or used, totally or in part, for non-commercial purposes provided that ECHA is acknowledged as the source: "Source: European Chemicals Agency, http://echa.europa.eu/". Such acknowledgement must be included in each copy of the material. ECHA permits and encourages organisations and individuals to create links to the ECHA website under the following cumulative conditions: Links can only be made to webpages that provide a link to the Legal Notice page. | |
Synthesis routes and methods
Procedure details
Retrosynthesis Analysis
AI-Powered Synthesis Planning: Our tool employs the Template_relevance Pistachio, Template_relevance Bkms_metabolic, Template_relevance Pistachio_ringbreaker, Template_relevance Reaxys, Template_relevance Reaxys_biocatalysis model, leveraging a vast database of chemical reactions to predict feasible synthetic routes.
One-Step Synthesis Focus: Specifically designed for one-step synthesis, it provides concise and direct routes for your target compounds, streamlining the synthesis process.
Accurate Predictions: Utilizing the extensive PISTACHIO, BKMS_METABOLIC, PISTACHIO_RINGBREAKER, REAXYS, REAXYS_BIOCATALYSIS database, our tool offers high-accuracy predictions, reflecting the latest in chemical research and data.
Strategy Settings
Precursor scoring | Relevance Heuristic |
---|---|
Min. plausibility | 0.01 |
Model | Template_relevance |
Template Set | Pistachio/Bkms_metabolic/Pistachio_ringbreaker/Reaxys/Reaxys_biocatalysis |
Top-N result to add to graph | 6 |
Feasible Synthetic Routes
Q & A
Q1: How does the incorporation of 4,6-difluoroindole into the structure of AMPK activators impact their pharmacokinetic properties compared to the parent 6-chloroindole core?
A1: [] Replacing the 6-chloroindole core with a this compound in a series of 5-aryl-indole-3-carboxylic acid derivatives led to improved metabolic stability. This modification also minimized the compounds' propensity for active transport by the renal organic anion transporter 3 (Oat3), ultimately resulting in lower renal clearance. This highlights the impact of subtle structural changes on the pharmacokinetic profile of drug candidates.
Disclaimer and Information on In-Vitro Research Products
Please be aware that all articles and product information presented on BenchChem are intended solely for informational purposes. The products available for purchase on BenchChem are specifically designed for in-vitro studies, which are conducted outside of living organisms. In-vitro studies, derived from the Latin term "in glass," involve experiments performed in controlled laboratory settings using cells or tissues. It is important to note that these products are not categorized as medicines or drugs, and they have not received approval from the FDA for the prevention, treatment, or cure of any medical condition, ailment, or disease. We must emphasize that any form of bodily introduction of these products into humans or animals is strictly prohibited by law. It is essential to adhere to these guidelines to ensure compliance with legal and ethical standards in research and experimentation.