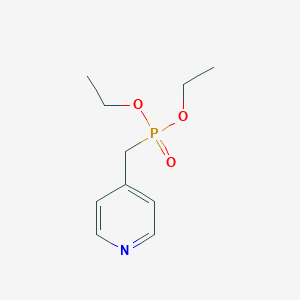
4-(Diethoxyphosphorylmethyl)pyridine
Overview
Description
4-(Diethoxyphosphorylmethyl)pyridine is an organic compound with the molecular formula C10H16NO3P and a molecular weight of 229.213 g/mol It is a derivative of pyridine, a basic heterocyclic organic compound, and contains a diethoxyphosphorylmethyl group attached to the fourth position of the pyridine ring
Preparation Methods
Synthetic Routes and Reaction Conditions
The synthesis of 4-(Diethoxyphosphorylmethyl)pyridine typically involves the reaction of pyridine with diethyl phosphite in the presence of a base such as sodium hydride or potassium tert-butoxide. The reaction is carried out under anhydrous conditions and at elevated temperatures to facilitate the formation of the desired product . The general reaction scheme is as follows:
Pyridine+Diethyl phosphiteBase, Heatthis compound
Industrial Production Methods
Industrial production methods for this compound are similar to laboratory synthesis but are scaled up to accommodate larger quantities. These methods often involve continuous flow reactors to ensure efficient mixing and heat transfer, which are crucial for maintaining reaction conditions and optimizing yield .
Chemical Reactions Analysis
Types of Reactions
4-(Diethoxyphosphorylmethyl)pyridine undergoes various chemical reactions, including:
Oxidation: The compound can be oxidized to form corresponding phosphonic acid derivatives.
Reduction: Reduction reactions can convert the phosphoryl group to a phosphine or phosphine oxide.
Substitution: The pyridine ring can undergo electrophilic and nucleophilic substitution reactions, allowing for further functionalization.
Common Reagents and Conditions
Oxidation: Common oxidizing agents include hydrogen peroxide and potassium permanganate.
Reduction: Reducing agents such as lithium aluminum hydride or sodium borohydride are used.
Substitution: Reagents like halogens, alkyl halides, and organometallic compounds are employed under various conditions.
Major Products Formed
The major products formed from these reactions depend on the specific reagents and conditions used. For example, oxidation typically yields phosphonic acids, while reduction can produce phosphines or phosphine oxides.
Scientific Research Applications
Hypolipidemic Effects
Research indicates that DEPM exhibits hypolipidemic properties, suggesting its potential in managing lipid disorders. The diethoxyphosphoryl group enhances its interaction with biological targets involved in lipid metabolism, making it a candidate for drug development aimed at treating conditions such as hyperlipidemia.
Antimicrobial Activity
DEPM has shown promising results as an antimicrobial agent. Preliminary studies indicate its toxicity against various bacterial strains, including Escherichia coli, which suggests potential applications in developing new antimicrobial drugs. The compound's mechanism may involve disrupting bacterial cell membranes or inhibiting essential metabolic pathways.
Anticancer Properties
Emerging research highlights the potential of DEPM derivatives in anticancer therapy. Compounds with similar structures have been evaluated for their cytotoxic effects against cancer cell lines, indicating that DEPM may also possess selective toxicity towards tumor cells while sparing normal cells .
Organic Synthesis and Catalysis
DEPM serves as a catalyst in numerous organic transformations due to its unique structure. It can facilitate reactions such as:
- Phosphonylation: Incorporating phosphonate groups into organic molecules.
- Cross-Coupling Reactions: Acting as a precursor for synthesizing complex organic compounds through palladium-catalyzed reactions.
The versatility of DEPM in catalysis is attributed to its ability to form strong bonds with metal ions and other biomolecules, influencing their reactivity .
Material Science Applications
In material science, DEPM is utilized in the synthesis of specialty chemicals and agrochemicals. Its phosphonate functionality allows it to form coordination complexes with metals, which can be applied in developing new materials with specific properties such as enhanced thermal stability or catalytic activity .
Case Study 1: Antimicrobial Efficacy
A study evaluated the antimicrobial activity of DEPM against E. coli strains. Results indicated that DEPM significantly inhibited bacterial growth at varying concentrations, demonstrating its potential as an effective antimicrobial agent .
Case Study 2: Hypolipidemic Activity
In another investigation, DEPM was tested for its effects on lipid profiles in animal models with induced hyperlipidemia. The results showed a marked reduction in total cholesterol and triglycerides, supporting its application in lipid management therapies.
Mechanism of Action
The mechanism of action of 4-(Diethoxyphosphorylmethyl)pyridine involves its interaction with various molecular targets. The phosphoryl group can participate in hydrogen bonding and coordination with metal ions, influencing the compound’s reactivity and biological activity. The pyridine ring can interact with nucleophiles and electrophiles, facilitating various chemical transformations .
Comparison with Similar Compounds
Similar Compounds
- Diethyl (4-pyridylmethyl)phosphonate
- Diethyl (pyridin-4-ylmethyl)phosphonate
- Phosphonic acid, P-(4-pyridinylmethyl)-, diethyl ester
Uniqueness
4-(Diethoxyphosphorylmethyl)pyridine is unique due to the specific positioning of the diethoxyphosphorylmethyl group on the pyridine ring, which imparts distinct chemical and physical properties.
Biological Activity
4-(Diethoxyphosphorylmethyl)pyridine (DEPM) is a chemical compound that has garnered attention for its diverse biological activities. This article explores its potential applications, mechanisms of action, and relevant research findings.
Chemical Structure and Properties
This compound has the molecular formula . It features a pyridine ring substituted with a diethoxyphosphorylmethyl group, which enhances its reactivity and biological properties. The phosphonate functionality of DEPM allows it to interact with various biological targets, potentially leading to therapeutic applications.
1. Antimicrobial Activity
Research indicates that DEPM exhibits notable antimicrobial properties . Preliminary studies have shown that it may be toxic against certain bacterial strains, including Escherichia coli. This suggests potential applications in developing new antimicrobial drugs.
2. Anticancer Properties
DEPM has also been studied for its anticancer potential . Investigations into its cytotoxic effects reveal that it may induce apoptosis in cancer cell lines, including human breast adenocarcinoma (MCF-7) and cervical carcinoma (HeLa) cells. The mechanism appears to involve the generation of reactive oxygen species (ROS), which are known to promote apoptosis .
3. Hypolipidemic Effects
Another area of interest is DEPM's potential hypolipidemic effects , which may involve modulation of lipid metabolism. This activity could have implications for managing lipid disorders and related conditions.
The biological activity of DEPM can be attributed to its interaction with various molecular targets:
- Hydrogen Bonding : The phosphoryl group can participate in hydrogen bonding, influencing the compound’s reactivity and biological activity.
- Coordination with Metal Ions : The diethoxyphosphoryl group may coordinate with metal ions, impacting enzymatic processes.
- Interaction with Nucleophiles and Electrophiles : The pyridine ring can engage in reactions with nucleophiles and electrophiles, facilitating diverse chemical transformations.
Comparative Analysis with Similar Compounds
To understand the uniqueness of DEPM, it is useful to compare it with structurally similar compounds:
Compound Name | Biological Activity | Notable Features |
---|---|---|
Diethyl (4-pyridylmethyl) phosphonate | Antimicrobial | Similar phosphonate structure |
Diethyl (pyridin-4-ylmethyl) phosphonate | Anticancer | Shares pyridine core |
Phosphonic acid, P-(4-pyridinylmethyl)-, diethyl ester | Lipid regulation | Potentially less reactive |
DEPM is distinct due to the specific positioning of the diethoxyphosphorylmethyl group on the pyridine ring, imparting unique chemical and physical properties compared to other similar compounds.
Case Studies and Research Findings
Several studies have highlighted the biological activity of DEPM:
- A study examining various organophosphonates found that DEPM exhibited significant antimicrobial activity against model strains of E. coli, indicating its potential as a new antimicrobial agent .
- Another investigation into the cytotoxic effects of DEPM on cancer cell lines demonstrated that it could induce apoptosis through ROS generation, comparable to established anticancer drugs like Doxorubicin .
Q & A
Basic Research Questions
Q. What are the common synthetic routes for 4-(Diethoxyphosphorylmethyl)pyridine, and how are purity and yield optimized?
The compound is typically synthesized via nucleophilic substitution or cross-coupling reactions. For example, Suzuki-Miyaura coupling (as seen in structurally similar pyridine derivatives) employs palladium catalysts, boronic acids, and halogenated precursors under inert conditions . Purification often involves column chromatography (silica gel) or recrystallization. Yield optimization requires careful control of reaction parameters (temperature, stoichiometry) and catalyst loading. Purity is assessed via HPLC or NMR .
Q. What spectroscopic methods are used to characterize this compound?
- NMR : and NMR are critical for confirming the phosphoryl-methyl group and pyridine ring substitution patterns. Large PH couplings (~60 Hz in similar phosphine complexes) help identify phosphorus-containing moieties .
- X-ray crystallography : Resolves molecular geometry and intermolecular interactions (e.g., π-π stacking, hydrogen bonding) in crystalline forms. For example, C–H⋯C interactions in pyridine derivatives stabilize crystal lattices .
- Mass spectrometry : Validates molecular weight and fragmentation patterns .
Q. What safety precautions are essential when handling this compound?
While toxicity data for this compound are limited, general pyridine derivative precautions apply:
- Use PPE (gloves, goggles) to avoid skin/eye contact .
- Work in a fume hood to prevent inhalation .
- Store in inert, dry conditions to avoid decomposition .
Advanced Research Questions
Q. How can computational modeling predict the reactivity of this compound in catalytic systems?
Density functional theory (DFT) simulations model electronic effects (e.g., phosphoryl group electron-withdrawing properties) on reaction pathways. For example, the phosphoryl group may stabilize transition states in cross-coupling reactions. Molecular docking studies can also predict binding affinities in biological applications .
Q. What strategies resolve contradictions between spectroscopic and crystallographic data?
Discrepancies may arise from dynamic effects (e.g., conformational flexibility in solution vs. solid-state rigidity). For example, NMR may indicate free rotation of the diethoxyphosphoryl group, while X-ray structures show fixed conformations. Multi-technique validation (e.g., variable-temperature NMR, Hirshfeld surface analysis) reconciles such differences .
Q. How is this compound functionalized for pharmaceutical intermediates?
- Nitration/Reduction : Nitration at the pyridine ring (using HNO/HSO) followed by reduction yields amino derivatives, which are precursors to quinolone antibiotics .
- Phosphoryl group modification : Hydrolysis of the diethoxy group generates phosphonic acids, useful in metal-organic frameworks (MOFs) or enzyme inhibitors .
Q. What analytical methods detect impurities in synthesized batches?
- HPLC-MS : Identifies trace organic impurities (e.g., unreacted precursors).
- NMR**: Detects phosphorylated byproducts.
- ICP-OES : Quantifies residual metal catalysts (e.g., Pd) .
Q. Methodological Challenges and Data Gaps
Q. How are stability studies designed for phosphorylated pyridines under varying conditions?
Accelerated degradation studies (e.g., exposure to heat, light, humidity) monitor decomposition via LC-MS. For example, hydrolytic stability of the P–O bond in aqueous buffers is assessed at different pH levels .
Q. Why is there limited ecological toxicity data, and how can researchers address this?
Current safety data sheets (SDS) often lack ecotoxicological profiles for pyridine derivatives . Standardized assays (e.g., Daphnia magna toxicity tests) and quantitative structure-activity relationship (QSAR) models can fill these gaps .
Properties
IUPAC Name |
4-(diethoxyphosphorylmethyl)pyridine | |
---|---|---|
Source | PubChem | |
URL | https://pubchem.ncbi.nlm.nih.gov | |
Description | Data deposited in or computed by PubChem | |
InChI |
InChI=1S/C10H16NO3P/c1-3-13-15(12,14-4-2)9-10-5-7-11-8-6-10/h5-8H,3-4,9H2,1-2H3 | |
Source | PubChem | |
URL | https://pubchem.ncbi.nlm.nih.gov | |
Description | Data deposited in or computed by PubChem | |
InChI Key |
IHGJNTGEJAJEKD-UHFFFAOYSA-N | |
Source | PubChem | |
URL | https://pubchem.ncbi.nlm.nih.gov | |
Description | Data deposited in or computed by PubChem | |
Canonical SMILES |
CCOP(=O)(CC1=CC=NC=C1)OCC | |
Source | PubChem | |
URL | https://pubchem.ncbi.nlm.nih.gov | |
Description | Data deposited in or computed by PubChem | |
Molecular Formula |
C10H16NO3P | |
Source | PubChem | |
URL | https://pubchem.ncbi.nlm.nih.gov | |
Description | Data deposited in or computed by PubChem | |
Molecular Weight |
229.21 g/mol | |
Source | PubChem | |
URL | https://pubchem.ncbi.nlm.nih.gov | |
Description | Data deposited in or computed by PubChem | |
Synthesis routes and methods
Procedure details
Disclaimer and Information on In-Vitro Research Products
Please be aware that all articles and product information presented on BenchChem are intended solely for informational purposes. The products available for purchase on BenchChem are specifically designed for in-vitro studies, which are conducted outside of living organisms. In-vitro studies, derived from the Latin term "in glass," involve experiments performed in controlled laboratory settings using cells or tissues. It is important to note that these products are not categorized as medicines or drugs, and they have not received approval from the FDA for the prevention, treatment, or cure of any medical condition, ailment, or disease. We must emphasize that any form of bodily introduction of these products into humans or animals is strictly prohibited by law. It is essential to adhere to these guidelines to ensure compliance with legal and ethical standards in research and experimentation.