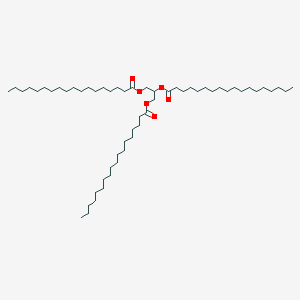
Tristearin
Overview
Description
It is an odorless, white powder that is insoluble in water but soluble in organic solvents like acetone and chloroform . Tristearin is commonly found in animal fats and some tropical plants such as palm. It is used in various industrial applications, including the production of biodiesel and as a model compound in scientific research .
Mechanism of Action
Target of Action
Tristearin, also known as glyceryl tristearate, is a triglyceride derived from three units of stearic acid . It primarily targets the lipid metabolism pathways in the body. It interacts with various enzymes involved in lipid metabolism, such as lipases, which are responsible for the breakdown of triglycerides .
Mode of Action
This compound is a type of fat that is broken down in the body by the action of enzymes called lipases. This process, known as lipolysis, converts this compound into glycerol and three molecules of stearic acid . The stearic acid can then be further metabolized or incorporated into other molecules. The breakdown of this compound can also lead to the production of energy in the form of ATP .
Biochemical Pathways
The primary biochemical pathway affected by this compound is lipid metabolism. Specifically, the breakdown of this compound can contribute to the process of beta-oxidation, where fatty acids are broken down to produce energy. Additionally, the stearic acid produced from this compound can be incorporated into phospholipids, which are essential components of cell membranes .
Pharmacokinetics
The pharmacokinetics of this compound, like other lipids, involves its digestion, absorption, distribution, metabolism, and excretion (ADME). This compound is insoluble in water and is digested in the gastrointestinal tract with the help of bile salts and lipases. The resulting monoglycerides and free fatty acids are then absorbed into the bloodstream. Once in the body, these molecules can be distributed to various tissues and organs where they can be metabolized or stored. The metabolites of this compound are eventually excreted from the body through the feces .
Result of Action
The breakdown of this compound results in the production of glycerol and stearic acid. These molecules can be used in various cellular processes. For example, glycerol can be used in the synthesis of glucose (a process called gluconeogenesis), while stearic acid can be used in the synthesis of other lipids or for energy production. Therefore, the action of this compound can influence cellular energy balance and lipid metabolism .
Action Environment
The action of this compound can be influenced by various environmental factors. For instance, the presence of other dietary fats can affect the rate of this compound digestion and absorption. Additionally, factors such as temperature can influence the physical state of this compound, which can impact its digestion and absorption . Furthermore, genetic factors can also influence how an individual metabolizes this compound .
Preparation Methods
Synthetic Routes and Reaction Conditions: Tristearin can be synthesized through the esterification of glycerol with stearic acid. The reaction typically involves heating glycerol and stearic acid in the presence of a catalyst, such as sulfuric acid, to facilitate the esterification process. The reaction is carried out at elevated temperatures to ensure complete conversion of the reactants to the triglyceride .
Industrial Production Methods: In industrial settings, this compound is often obtained through the interesterification of fats and oils. This process involves the rearrangement of fatty acids on the glycerol backbone, exploiting the higher melting point of this compound to separate it from other triglycerides. Physical vapor deposition and coaxial electrospray processing are also used to prepare this compound for specific applications .
Types of Reactions:
Transesterification: this compound undergoes transesterification reactions with alcohols, such as methanol, in the presence of a catalyst to produce biodiesel.
Common Reagents and Conditions:
Transesterification: Methanol, sodium hydroxide, basified polyacrylonitrile fibers, temperatures around 65°C.
Hydrolysis: Water, sodium hydroxide, elevated temperatures.
Major Products:
Transesterification: Methyl stearate (biodiesel), glycerol.
Hydrolysis: Glycerol, stearic acid.
Scientific Research Applications
Tristearin is widely used in scientific research due to its well-characterized properties and availability. Some of its applications include:
Comparison with Similar Compounds
Triolein: A triglyceride composed of glycerol and three oleic acid molecules.
Tripalmitin: A triglyceride composed of glycerol and three palmitic acid molecules.
Uniqueness: Tristearin’s high melting point and crystalline structure make it suitable for applications requiring solid lipid matrices, such as in drug delivery and as a model compound for studying lipid crystallization .
Properties
IUPAC Name |
2,3-di(octadecanoyloxy)propyl octadecanoate | |
---|---|---|
Source | PubChem | |
URL | https://pubchem.ncbi.nlm.nih.gov | |
Description | Data deposited in or computed by PubChem | |
InChI |
InChI=1S/C57H110O6/c1-4-7-10-13-16-19-22-25-28-31-34-37-40-43-46-49-55(58)61-52-54(63-57(60)51-48-45-42-39-36-33-30-27-24-21-18-15-12-9-6-3)53-62-56(59)50-47-44-41-38-35-32-29-26-23-20-17-14-11-8-5-2/h54H,4-53H2,1-3H3 | |
Source | PubChem | |
URL | https://pubchem.ncbi.nlm.nih.gov | |
Description | Data deposited in or computed by PubChem | |
InChI Key |
DCXXMTOCNZCJGO-UHFFFAOYSA-N | |
Source | PubChem | |
URL | https://pubchem.ncbi.nlm.nih.gov | |
Description | Data deposited in or computed by PubChem | |
Canonical SMILES |
CCCCCCCCCCCCCCCCCC(=O)OCC(COC(=O)CCCCCCCCCCCCCCCCC)OC(=O)CCCCCCCCCCCCCCCCC | |
Source | PubChem | |
URL | https://pubchem.ncbi.nlm.nih.gov | |
Description | Data deposited in or computed by PubChem | |
Molecular Formula |
C57H110O6 | |
Source | PubChem | |
URL | https://pubchem.ncbi.nlm.nih.gov | |
Description | Data deposited in or computed by PubChem | |
DSSTOX Substance ID |
DTXSID8047503 | |
Record name | Glyceryl tristearate | |
Source | EPA DSSTox | |
URL | https://comptox.epa.gov/dashboard/DTXSID8047503 | |
Description | DSSTox provides a high quality public chemistry resource for supporting improved predictive toxicology. | |
Molecular Weight |
891.5 g/mol | |
Source | PubChem | |
URL | https://pubchem.ncbi.nlm.nih.gov | |
Description | Data deposited in or computed by PubChem | |
Physical Description |
Dry Powder; Other Solid, Colorless or white odorless solid; [HSDB] White powder; [MSDSonline], Solid | |
Record name | Octadecanoic acid, 1,1',1''-(1,2,3-propanetriyl) ester | |
Source | EPA Chemicals under the TSCA | |
URL | https://www.epa.gov/chemicals-under-tsca | |
Description | EPA Chemicals under the Toxic Substances Control Act (TSCA) collection contains information on chemicals and their regulations under TSCA, including non-confidential content from the TSCA Chemical Substance Inventory and Chemical Data Reporting. | |
Record name | Tristearin | |
Source | Haz-Map, Information on Hazardous Chemicals and Occupational Diseases | |
URL | https://haz-map.com/Agents/7659 | |
Description | Haz-Map® is an occupational health database designed for health and safety professionals and for consumers seeking information about the adverse effects of workplace exposures to chemical and biological agents. | |
Explanation | Copyright (c) 2022 Haz-Map(R). All rights reserved. Unless otherwise indicated, all materials from Haz-Map are copyrighted by Haz-Map(R). No part of these materials, either text or image may be used for any purpose other than for personal use. Therefore, reproduction, modification, storage in a retrieval system or retransmission, in any form or by any means, electronic, mechanical or otherwise, for reasons other than personal use, is strictly prohibited without prior written permission. | |
Record name | TG(18:0/18:0/18:0) | |
Source | Human Metabolome Database (HMDB) | |
URL | http://www.hmdb.ca/metabolites/HMDB0005393 | |
Description | The Human Metabolome Database (HMDB) is a freely available electronic database containing detailed information about small molecule metabolites found in the human body. | |
Explanation | HMDB is offered to the public as a freely available resource. Use and re-distribution of the data, in whole or in part, for commercial purposes requires explicit permission of the authors and explicit acknowledgment of the source material (HMDB) and the original publication (see the HMDB citing page). We ask that users who download significant portions of the database cite the HMDB paper in any resulting publications. | |
Solubility |
Insoluble in water. Soluble in chloroform, carbon disulfide, Insoluble in ethanol; very soluble in acetone, benzene, Soluble in hot alcohol; almost insoluble in cold alcohol, ether, petroleum ether | |
Record name | TRISTEARIN | |
Source | Hazardous Substances Data Bank (HSDB) | |
URL | https://pubchem.ncbi.nlm.nih.gov/source/hsdb/5690 | |
Description | The Hazardous Substances Data Bank (HSDB) is a toxicology database that focuses on the toxicology of potentially hazardous chemicals. It provides information on human exposure, industrial hygiene, emergency handling procedures, environmental fate, regulatory requirements, nanomaterials, and related areas. The information in HSDB has been assessed by a Scientific Review Panel. | |
Density |
0.8559 at 90 °C/4 °C | |
Record name | TRISTEARIN | |
Source | Hazardous Substances Data Bank (HSDB) | |
URL | https://pubchem.ncbi.nlm.nih.gov/source/hsdb/5690 | |
Description | The Hazardous Substances Data Bank (HSDB) is a toxicology database that focuses on the toxicology of potentially hazardous chemicals. It provides information on human exposure, industrial hygiene, emergency handling procedures, environmental fate, regulatory requirements, nanomaterials, and related areas. The information in HSDB has been assessed by a Scientific Review Panel. | |
Vapor Pressure |
5.4X10-17 mm Hg at 25 °C /Estimated/ | |
Record name | TRISTEARIN | |
Source | Hazardous Substances Data Bank (HSDB) | |
URL | https://pubchem.ncbi.nlm.nih.gov/source/hsdb/5690 | |
Description | The Hazardous Substances Data Bank (HSDB) is a toxicology database that focuses on the toxicology of potentially hazardous chemicals. It provides information on human exposure, industrial hygiene, emergency handling procedures, environmental fate, regulatory requirements, nanomaterials, and related areas. The information in HSDB has been assessed by a Scientific Review Panel. | |
Color/Form |
White powder, Colorless crystals or powder | |
CAS No. |
555-43-1, 68334-00-9 | |
Record name | Tristearin | |
Source | CAS Common Chemistry | |
URL | https://commonchemistry.cas.org/detail?cas_rn=555-43-1 | |
Description | CAS Common Chemistry is an open community resource for accessing chemical information. Nearly 500,000 chemical substances from CAS REGISTRY cover areas of community interest, including common and frequently regulated chemicals, and those relevant to high school and undergraduate chemistry classes. This chemical information, curated by our expert scientists, is provided in alignment with our mission as a division of the American Chemical Society. | |
Explanation | The data from CAS Common Chemistry is provided under a CC-BY-NC 4.0 license, unless otherwise stated. | |
Record name | Tristearin | |
Source | ChemIDplus | |
URL | https://pubchem.ncbi.nlm.nih.gov/substance/?source=chemidplus&sourceid=0000555431 | |
Description | ChemIDplus is a free, web search system that provides access to the structure and nomenclature authority files used for the identification of chemical substances cited in National Library of Medicine (NLM) databases, including the TOXNET system. | |
Record name | Octadecanoic acid, 1,1',1''-(1,2,3-propanetriyl) ester | |
Source | EPA Chemicals under the TSCA | |
URL | https://www.epa.gov/chemicals-under-tsca | |
Description | EPA Chemicals under the Toxic Substances Control Act (TSCA) collection contains information on chemicals and their regulations under TSCA, including non-confidential content from the TSCA Chemical Substance Inventory and Chemical Data Reporting. | |
Record name | Glyceryl tristearate | |
Source | EPA DSSTox | |
URL | https://comptox.epa.gov/dashboard/DTXSID8047503 | |
Description | DSSTox provides a high quality public chemistry resource for supporting improved predictive toxicology. | |
Record name | Glycerol tristearate | |
Source | European Chemicals Agency (ECHA) | |
URL | https://echa.europa.eu/substance-information/-/substanceinfo/100.008.271 | |
Description | The European Chemicals Agency (ECHA) is an agency of the European Union which is the driving force among regulatory authorities in implementing the EU's groundbreaking chemicals legislation for the benefit of human health and the environment as well as for innovation and competitiveness. | |
Explanation | Use of the information, documents and data from the ECHA website is subject to the terms and conditions of this Legal Notice, and subject to other binding limitations provided for under applicable law, the information, documents and data made available on the ECHA website may be reproduced, distributed and/or used, totally or in part, for non-commercial purposes provided that ECHA is acknowledged as the source: "Source: European Chemicals Agency, http://echa.europa.eu/". Such acknowledgement must be included in each copy of the material. ECHA permits and encourages organisations and individuals to create links to the ECHA website under the following cumulative conditions: Links can only be made to webpages that provide a link to the Legal Notice page. | |
Record name | Cottonseed oil, hydrogenated | |
Source | European Chemicals Agency (ECHA) | |
URL | https://echa.europa.eu/substance-information/-/substanceinfo/100.063.439 | |
Description | The European Chemicals Agency (ECHA) is an agency of the European Union which is the driving force among regulatory authorities in implementing the EU's groundbreaking chemicals legislation for the benefit of human health and the environment as well as for innovation and competitiveness. | |
Explanation | Use of the information, documents and data from the ECHA website is subject to the terms and conditions of this Legal Notice, and subject to other binding limitations provided for under applicable law, the information, documents and data made available on the ECHA website may be reproduced, distributed and/or used, totally or in part, for non-commercial purposes provided that ECHA is acknowledged as the source: "Source: European Chemicals Agency, http://echa.europa.eu/". Such acknowledgement must be included in each copy of the material. ECHA permits and encourages organisations and individuals to create links to the ECHA website under the following cumulative conditions: Links can only be made to webpages that provide a link to the Legal Notice page. | |
Record name | GLYCERYL TRISTEARATE | |
Source | FDA Global Substance Registration System (GSRS) | |
URL | https://gsrs.ncats.nih.gov/ginas/app/beta/substances/P6OCJ2551R | |
Description | The FDA Global Substance Registration System (GSRS) enables the efficient and accurate exchange of information on what substances are in regulated products. Instead of relying on names, which vary across regulatory domains, countries, and regions, the GSRS knowledge base makes it possible for substances to be defined by standardized, scientific descriptions. | |
Explanation | Unless otherwise noted, the contents of the FDA website (www.fda.gov), both text and graphics, are not copyrighted. They are in the public domain and may be republished, reprinted and otherwise used freely by anyone without the need to obtain permission from FDA. Credit to the U.S. Food and Drug Administration as the source is appreciated but not required. | |
Record name | TRISTEARIN | |
Source | Hazardous Substances Data Bank (HSDB) | |
URL | https://pubchem.ncbi.nlm.nih.gov/source/hsdb/5690 | |
Description | The Hazardous Substances Data Bank (HSDB) is a toxicology database that focuses on the toxicology of potentially hazardous chemicals. It provides information on human exposure, industrial hygiene, emergency handling procedures, environmental fate, regulatory requirements, nanomaterials, and related areas. The information in HSDB has been assessed by a Scientific Review Panel. | |
Melting Point |
MP: 55 °C (ALPHA), 64.5 °C (BETA'), 73 °C (BETA) | |
Record name | TRISTEARIN | |
Source | Hazardous Substances Data Bank (HSDB) | |
URL | https://pubchem.ncbi.nlm.nih.gov/source/hsdb/5690 | |
Description | The Hazardous Substances Data Bank (HSDB) is a toxicology database that focuses on the toxicology of potentially hazardous chemicals. It provides information on human exposure, industrial hygiene, emergency handling procedures, environmental fate, regulatory requirements, nanomaterials, and related areas. The information in HSDB has been assessed by a Scientific Review Panel. | |
Synthesis routes and methods I
Procedure details
Synthesis routes and methods II
Procedure details
Synthesis routes and methods III
Procedure details
Synthesis routes and methods IV
Procedure details
Retrosynthesis Analysis
AI-Powered Synthesis Planning: Our tool employs the Template_relevance Pistachio, Template_relevance Bkms_metabolic, Template_relevance Pistachio_ringbreaker, Template_relevance Reaxys, Template_relevance Reaxys_biocatalysis model, leveraging a vast database of chemical reactions to predict feasible synthetic routes.
One-Step Synthesis Focus: Specifically designed for one-step synthesis, it provides concise and direct routes for your target compounds, streamlining the synthesis process.
Accurate Predictions: Utilizing the extensive PISTACHIO, BKMS_METABOLIC, PISTACHIO_RINGBREAKER, REAXYS, REAXYS_BIOCATALYSIS database, our tool offers high-accuracy predictions, reflecting the latest in chemical research and data.
Strategy Settings
Precursor scoring | Relevance Heuristic |
---|---|
Min. plausibility | 0.01 |
Model | Template_relevance |
Template Set | Pistachio/Bkms_metabolic/Pistachio_ringbreaker/Reaxys/Reaxys_biocatalysis |
Top-N result to add to graph | 6 |
Feasible Synthetic Routes
Q1: What is the molecular formula and weight of tristearin?
A1: this compound, also known as glyceryl tristearate, has a molecular formula of C57H110O6 and a molecular weight of 891.49 g/mol.
Q2: What spectroscopic data is available for characterizing this compound?
A2: Several spectroscopic techniques are employed to characterize this compound, including:
- X-ray Diffraction (XRD): Used to determine the crystalline structure and polymorphic forms of this compound. [, , , , , ]
- Differential Scanning Calorimetry (DSC): Provides information about melting points, enthalpies of fusion, and polymorphic transitions. [, , , , , , , ]
- Nuclear Magnetic Resonance (NMR): Useful for structural elucidation and studying molecular interactions. []
- Fourier Transform Infrared Spectroscopy (FT-IR): Detects functional groups and interactions between this compound and other molecules. []
Q3: How does this compound behave under different environmental conditions?
A: this compound exhibits polymorphism, meaning it can exist in different crystalline forms (α, β', and β) with distinct physical properties. These forms interconvert depending on factors like temperature and cooling rate. [, , , ] The stability of each form is influenced by storage temperature, with higher temperatures promoting transitions to more stable forms. []
Q4: Are there ways to control this compound's polymorphic behavior?
A: Yes, the addition of liquid lipids like isopropyl myristate and ethyl oleate can act as polymorphic modifiers, promoting the formation of the stable β-form. [] Emulsifiers such as sorbitan monostearate and glycerol-l-stearate can also influence the crystal lattice parameters of this compound, potentially impacting its melting point and other properties. []
Q5: How does particle size affect the compression behavior of this compound powder?
A: The energy required to consolidate this compound powder decreases with increasing environmental temperature. Higher temperatures lower the yielding stress of this compound particles, enhancing plastic deformation. [] Interestingly, changes in crystal structure seem to depend more on particle size than temperature. []
Q6: How is this compound used in drug delivery systems?
A: this compound is investigated as a lipid matrix for solid lipid nanoparticles (SLNs), which can encapsulate and deliver drugs. [, , , ] Its ability to exist in different polymorphic forms influences drug loading, release kinetics, and stability. [] this compound SLNs have shown promise in enhancing the bioavailability and therapeutic efficiency of drugs like celecoxib for treating rheumatoid arthritis. []
Q7: How does this compound interact with other components in formulations?
A: Research suggests a synergistic increase in interfacial shear viscosity when this compound crystals are present in oil phases alongside proteins like lysozyme and sodium caseinate in aqueous phases. This phenomenon is attributed to the adsorption of hydrophobic protein groups onto the this compound crystals. [, ]
Q8: How does this compound impact the absorption of other lipids?
A: Animal studies show that the presence of this compound in the diet can significantly reduce cholesterol absorption compared to other triglycerides like tripalmitin or triolein. [, ] This effect is partly attributed to the lower absorption rate of stearic acid, a primary component of this compound. []
Q9: Can this compound be used to study cuticle interactions?
A: Yes, this compound has been proposed as a model cuticle for studying agricultural adjuvant systems. Its well-characterized crystalline structure and behavior make it suitable for screening adjuvants for their ability to disrupt cuticle barriers. []
Q10: Have computational methods been used to study this compound?
A: Yes, molecular dynamics simulations have been employed to understand the behavior of triolein, another triglyceride, in nanoscale confinements between this compound crystalline nanoplatelets (CNPs). These simulations help define oil-binding capacities and could be valuable for designing healthier food products. []
Q11: What other computational approaches have been applied to this compound research?
A: Dissipative Particle Dynamics (DPD) simulations have been utilized to investigate the compatibility and distribution of ibuprofen within various carrier materials, including this compound. These simulations offer insights into drug release kinetics and the suitability of different carriers for specific drug delivery applications. []
Disclaimer and Information on In-Vitro Research Products
Please be aware that all articles and product information presented on BenchChem are intended solely for informational purposes. The products available for purchase on BenchChem are specifically designed for in-vitro studies, which are conducted outside of living organisms. In-vitro studies, derived from the Latin term "in glass," involve experiments performed in controlled laboratory settings using cells or tissues. It is important to note that these products are not categorized as medicines or drugs, and they have not received approval from the FDA for the prevention, treatment, or cure of any medical condition, ailment, or disease. We must emphasize that any form of bodily introduction of these products into humans or animals is strictly prohibited by law. It is essential to adhere to these guidelines to ensure compliance with legal and ethical standards in research and experimentation.