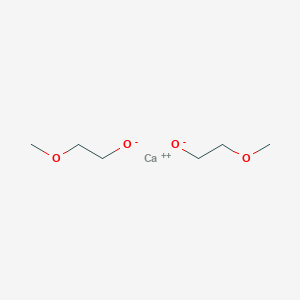
calcium methoxyethoxide
Overview
Description
calcium methoxyethoxide is an organometallic compound with the molecular formula C6H14CaO4.
Preparation Methods
Synthetic Routes and Reaction Conditions
calcium methoxyethoxide can be synthesized through a hydrothermal process. This method involves reacting calcium oxide (CaO) with methoxyethanol under controlled temperature and pressure conditions. The reaction typically occurs at elevated temperatures to ensure complete conversion .
Industrial Production Methods
In industrial settings, calcium 2-methoxyethanolate is produced using a similar hydrothermal process but on a larger scale. The process involves the use of high-purity calcium oxide and methoxyethanol, with the reaction being carried out in specialized reactors to maintain the required temperature and pressure conditions .
Chemical Reactions Analysis
Types of Reactions
calcium methoxyethoxide undergoes various chemical reactions, including:
Oxidation: Reacts with oxygen to form calcium oxide and methoxyethanol.
Reduction: Can be reduced to calcium metal under specific conditions.
Substitution: Participates in substitution reactions where the methoxyethanol group is replaced by other functional groups.
Common Reagents and Conditions
Oxidation: Requires an oxidizing agent such as oxygen or hydrogen peroxide.
Reduction: Involves reducing agents like lithium aluminum hydride.
Substitution: Utilizes reagents like halogens or other nucleophiles.
Major Products
Oxidation: Produces calcium oxide and methoxyethanol.
Reduction: Yields calcium metal.
Substitution: Forms various substituted calcium compounds depending on the reagents used.
Scientific Research Applications
calcium methoxyethoxide has several applications in scientific research:
Chemistry: Used as a catalyst in organic synthesis and as a precursor for other calcium-based compounds.
Medicine: Explored for its potential use in drug delivery systems and as a calcium supplement.
Industry: Utilized in the production of biodiesel and as a catalyst in various industrial processes.
Mechanism of Action
calcium methoxyethoxide exerts its effects through its interaction with calcium ion channels and signaling pathways. It can modulate calcium levels within cells, influencing various cellular processes such as muscle contraction, neurotransmitter release, and enzyme activity . The compound’s ability to release calcium ions makes it a valuable tool in studying calcium-dependent biological processes .
Comparison with Similar Compounds
Similar Compounds
Calcium methoxide: Similar in structure but lacks the methoxyethanol group.
Calcium ethoxide: Contains an ethoxide group instead of methoxyethanol.
Calcium isopropoxide: Features an isopropoxide group instead of methoxyethanol.
Uniqueness
calcium methoxyethoxide is unique due to its methoxyethanol group, which imparts distinct chemical properties and reactivity. This makes it particularly useful in specific applications where other calcium alkoxides may not be as effective .
Properties
IUPAC Name |
calcium;2-methoxyethanolate | |
---|---|---|
Source | PubChem | |
URL | https://pubchem.ncbi.nlm.nih.gov | |
Description | Data deposited in or computed by PubChem | |
InChI |
InChI=1S/2C3H7O2.Ca/c2*1-5-3-2-4;/h2*2-3H2,1H3;/q2*-1;+2 | |
Source | PubChem | |
URL | https://pubchem.ncbi.nlm.nih.gov | |
Description | Data deposited in or computed by PubChem | |
InChI Key |
OFPISIUOJVZBMS-UHFFFAOYSA-N | |
Source | PubChem | |
URL | https://pubchem.ncbi.nlm.nih.gov | |
Description | Data deposited in or computed by PubChem | |
Canonical SMILES |
COCC[O-].COCC[O-].[Ca+2] | |
Source | PubChem | |
URL | https://pubchem.ncbi.nlm.nih.gov | |
Description | Data deposited in or computed by PubChem | |
Molecular Formula |
C6H14CaO4 | |
Source | PubChem | |
URL | https://pubchem.ncbi.nlm.nih.gov | |
Description | Data deposited in or computed by PubChem | |
Related CAS |
109-86-4 (Parent) | |
Record name | Ethanol, 2-methoxy-, calcium salt (2:1) | |
Source | ChemIDplus | |
URL | https://pubchem.ncbi.nlm.nih.gov/substance/?source=chemidplus&sourceid=0028099674 | |
Description | ChemIDplus is a free, web search system that provides access to the structure and nomenclature authority files used for the identification of chemical substances cited in National Library of Medicine (NLM) databases, including the TOXNET system. | |
DSSTOX Substance ID |
DTXSID10890813 | |
Record name | Ethanol, 2-methoxy-, calcium salt (2:1) | |
Source | EPA DSSTox | |
URL | https://comptox.epa.gov/dashboard/DTXSID10890813 | |
Description | DSSTox provides a high quality public chemistry resource for supporting improved predictive toxicology. | |
Molecular Weight |
190.25 g/mol | |
Source | PubChem | |
URL | https://pubchem.ncbi.nlm.nih.gov | |
Description | Data deposited in or computed by PubChem | |
CAS No. |
28099-67-4 | |
Record name | Ethanol, 2-methoxy-, calcium salt (2:1) | |
Source | ChemIDplus | |
URL | https://pubchem.ncbi.nlm.nih.gov/substance/?source=chemidplus&sourceid=0028099674 | |
Description | ChemIDplus is a free, web search system that provides access to the structure and nomenclature authority files used for the identification of chemical substances cited in National Library of Medicine (NLM) databases, including the TOXNET system. | |
Record name | Ethanol, 2-methoxy-, calcium salt (2:1) | |
Source | EPA Chemicals under the TSCA | |
URL | https://www.epa.gov/chemicals-under-tsca | |
Description | EPA Chemicals under the Toxic Substances Control Act (TSCA) collection contains information on chemicals and their regulations under TSCA, including non-confidential content from the TSCA Chemical Substance Inventory and Chemical Data Reporting. | |
Record name | Ethanol, 2-methoxy-, calcium salt (2:1) | |
Source | EPA DSSTox | |
URL | https://comptox.epa.gov/dashboard/DTXSID10890813 | |
Description | DSSTox provides a high quality public chemistry resource for supporting improved predictive toxicology. | |
Record name | Calcium 2-methoxyethanolate | |
Source | European Chemicals Agency (ECHA) | |
URL | https://echa.europa.eu/substance-information/-/substanceinfo/100.044.386 | |
Description | The European Chemicals Agency (ECHA) is an agency of the European Union which is the driving force among regulatory authorities in implementing the EU's groundbreaking chemicals legislation for the benefit of human health and the environment as well as for innovation and competitiveness. | |
Explanation | Use of the information, documents and data from the ECHA website is subject to the terms and conditions of this Legal Notice, and subject to other binding limitations provided for under applicable law, the information, documents and data made available on the ECHA website may be reproduced, distributed and/or used, totally or in part, for non-commercial purposes provided that ECHA is acknowledged as the source: "Source: European Chemicals Agency, http://echa.europa.eu/". Such acknowledgement must be included in each copy of the material. ECHA permits and encourages organisations and individuals to create links to the ECHA website under the following cumulative conditions: Links can only be made to webpages that provide a link to the Legal Notice page. | |
Q1: What is the role of Calcium 2-methoxyethoxide in the synthesis of calcium phosphate glass nanoparticles?
A1: Calcium 2-methoxyethoxide serves as a precursor in the sol-gel synthesis of binary (P205-Ca0) calcium phosphate glass (CPg) nanoparticles []. In this process, it reacts with ethylphosphate in an ethanolic medium, with ammonia (NH3(aq)) acting as a catalyst. This reaction leads to the formation of a mixture primarily composed of amorphous calcium monoethylphosphate, alongside byproducts like portlandite (in the presence of excess calcium) or NH4H2P04 (in the absence of calcium) []. Subsequent thermal treatment transforms these initial products into more stable but still degradable inorganic forms, including amorphous calcium trimetaphosphate (ACTMP), pyrophosphate (ACPP), orthophosphate (ACP), and calcite, depending on the calcium content and the temperature applied [].
Disclaimer and Information on In-Vitro Research Products
Please be aware that all articles and product information presented on BenchChem are intended solely for informational purposes. The products available for purchase on BenchChem are specifically designed for in-vitro studies, which are conducted outside of living organisms. In-vitro studies, derived from the Latin term "in glass," involve experiments performed in controlled laboratory settings using cells or tissues. It is important to note that these products are not categorized as medicines or drugs, and they have not received approval from the FDA for the prevention, treatment, or cure of any medical condition, ailment, or disease. We must emphasize that any form of bodily introduction of these products into humans or animals is strictly prohibited by law. It is essential to adhere to these guidelines to ensure compliance with legal and ethical standards in research and experimentation.