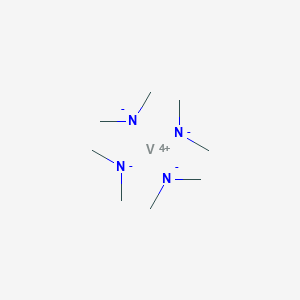
Vanadium tetrakis(dimethylamide)
- Click on QUICK INQUIRY to receive a quote from our team of experts.
- With the quality product at a COMPETITIVE price, you can focus more on your research.
Overview
Description
Vanadium tetrakis(dimethylamide), also known as Tetrakis(dimethylamino)vanadium, is a compound with the molecular formula C10H30N5V and a molecular weight of 271.32 . It is used for research and development purposes .
Molecular Structure Analysis
The compound has non-crystallographic D2d molecular symmetry and contains an approximately tetrahedrally coordinated V atom with dimethylamido ligands . Each Nitrogen atom features a nearly trigonal planar geometry .Chemical Reactions Analysis
While specific chemical reactions involving Vanadium tetrakis(dimethylamide) are not detailed in the retrieved sources, vanadium compounds have been studied for their reactions, including oxidations of alkanes, alkenes, arenes, alcohols, aldehydes, ketones, and sulfur species, as well as oxidative C–C and C–O bond cleavage, carbon–carbon bond formation, deoxydehydration, haloperoxidase, cyanation .Scientific Research Applications
I have conducted a search for the scientific research applications of Vanadium tetrakis(dimethylamide), but the information available is limited. The primary application mentioned is its use as a precursor in Atomic Layer Deposition (ALD) of vanadium oxide thin films, which is a technique used to deposit thin films of material onto a substrate with high precision .
Other potential applications such as battery materials, gas sensing, data storage, optical computing, and infrared modulators in missile guidance systems are mentioned, but these are applications of vanadium oxide thin films rather than Vanadium tetrakis(dimethylamide) itself .
Safety And Hazards
Safety data sheets suggest avoiding dust formation, breathing mist, gas or vapours, and contact with skin and eye when handling Vanadium tetrakis(dimethylamide) . In case of inhalation, move the victim into fresh air and give artificial respiration if necessary . In case of skin contact, take off contaminated clothing immediately and wash off with soap and plenty of water . In case of ingestion, rinse mouth with water and do not induce vomiting .
properties
IUPAC Name |
dimethylazanide;vanadium(4+) |
Source
|
---|---|---|
Source | PubChem | |
URL | https://pubchem.ncbi.nlm.nih.gov | |
Description | Data deposited in or computed by PubChem | |
InChI |
InChI=1S/4C2H6N.V/c4*1-3-2;/h4*1-2H3;/q4*-1;+4 |
Source
|
Source | PubChem | |
URL | https://pubchem.ncbi.nlm.nih.gov | |
Description | Data deposited in or computed by PubChem | |
InChI Key |
PALZAXLZULBBHA-UHFFFAOYSA-N |
Source
|
Source | PubChem | |
URL | https://pubchem.ncbi.nlm.nih.gov | |
Description | Data deposited in or computed by PubChem | |
Canonical SMILES |
C[N-]C.C[N-]C.C[N-]C.C[N-]C.[V+4] |
Source
|
Source | PubChem | |
URL | https://pubchem.ncbi.nlm.nih.gov | |
Description | Data deposited in or computed by PubChem | |
Molecular Formula |
C8H24N4V |
Source
|
Source | PubChem | |
URL | https://pubchem.ncbi.nlm.nih.gov | |
Description | Data deposited in or computed by PubChem | |
DSSTOX Substance ID |
DTXSID40173545 |
Source
|
Record name | Tetrakis(dimethylamino)vanadium | |
Source | EPA DSSTox | |
URL | https://comptox.epa.gov/dashboard/DTXSID40173545 | |
Description | DSSTox provides a high quality public chemistry resource for supporting improved predictive toxicology. | |
Molecular Weight |
227.25 g/mol |
Source
|
Source | PubChem | |
URL | https://pubchem.ncbi.nlm.nih.gov | |
Description | Data deposited in or computed by PubChem | |
Product Name |
Vanadium tetrakis(dimethylamide) | |
CAS RN |
19824-56-7 |
Source
|
Record name | Tetrakis(dimethylamino)vanadium | |
Source | EPA DSSTox | |
URL | https://comptox.epa.gov/dashboard/DTXSID40173545 | |
Description | DSSTox provides a high quality public chemistry resource for supporting improved predictive toxicology. | |
Disclaimer and Information on In-Vitro Research Products
Please be aware that all articles and product information presented on BenchChem are intended solely for informational purposes. The products available for purchase on BenchChem are specifically designed for in-vitro studies, which are conducted outside of living organisms. In-vitro studies, derived from the Latin term "in glass," involve experiments performed in controlled laboratory settings using cells or tissues. It is important to note that these products are not categorized as medicines or drugs, and they have not received approval from the FDA for the prevention, treatment, or cure of any medical condition, ailment, or disease. We must emphasize that any form of bodily introduction of these products into humans or animals is strictly prohibited by law. It is essential to adhere to these guidelines to ensure compliance with legal and ethical standards in research and experimentation.