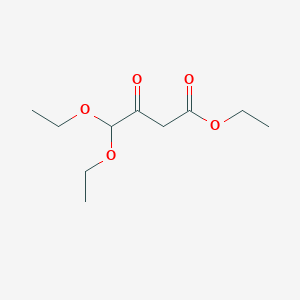
Ethyl 4,4-diethoxy-3-oxobutanoate
Overview
Description
Ethyl 4,4-diethoxy-3-oxobutanoate is a chemical compound with the molecular formula C10H18O5 . It is used as an intermediate in the synthesis of pharmaceuticals .
Molecular Structure Analysis
The molecular structure of Ethyl 4,4-diethoxy-3-oxobutanoate is represented by the SMILES stringO=C(CC(OCC)=O)C(OCC)OCC
. It has an empirical formula of C10H18O5 and a molecular weight of 218.25 . Physical And Chemical Properties Analysis
Ethyl 4,4-diethoxy-3-oxobutanoate is a liquid at room temperature . It has a molecular weight of 218.25 . The compound’s density, boiling point, and other physical properties are not detailed in the search results.Scientific Research Applications
Synthesis of Trifluoromethyl Heterocycles
Ethyl 4,4-diethoxy-3-oxobutanoate is used as an intermediate in the synthesis of various trifluoromethyl heterocycles. This synthesis involves rhodium(II) or copper(II) catalyzed carbene X-H insertion reactions, enabling the creation of trifluoromethyl-oxazoles, -thiazoles, -imidazoles, -1,2,4-triazines, and -pyridines from the diazoketoester (Honey et al., 2012).
Reactions with Malonate and Malononitrile
Ethyl 4,4-diethoxy-3-oxobutanoate reacts with diethyl malonate, methyl cyanoacetate, and malononitrile to produce various compounds. These reactions yield products like ethyl 5-ethoxycarbonyl-3-oxohexanedioate and ethyl 5-amino-4-cyanofuran-2-acetate, indicating its utility in diverse chemical syntheses (Kato et al., 1978).
Enzyme-Catalyzed Asymmetric Reduction
In a study, ethyl 4,4-diethoxy-3-oxobutanoate underwent enzyme-catalyzed asymmetric reduction in an organic solvent-water diphasic system. This process used NADPH-dependent aldehyde reductase and glucose dehydrogenase for the reduction, highlighting its potential in biocatalysis and organic synthesis (Shimizu et al., 1990).
Antioxidant Properties of Derivatives
4-Hydroxycoumarin derivatives, including ethyl 4,4-diethoxy-3-oxobutanoate, have been investigated for their antioxidant properties. These compounds, tested in vitro, showed potential as free radical scavengers, which could be significant in developing new antioxidant agents (Stanchev et al., 2009).
Synthesis of 4H-Pyran Derivatives
Ethyl 4,4-diethoxy-3-oxobutanoate is involved in synthesizing diethyl 4-oxo-4H-pyran-2,5-dicarboxylate, a key intermediate in producing various pyran and pyridine derivatives. This synthesis pathway offers new routes for creating compounds with potential pharmacological applications (Obydennov et al., 2013).
Safety And Hazards
Future Directions
properties
IUPAC Name |
ethyl 4,4-diethoxy-3-oxobutanoate | |
---|---|---|
Source | PubChem | |
URL | https://pubchem.ncbi.nlm.nih.gov | |
Description | Data deposited in or computed by PubChem | |
InChI |
InChI=1S/C10H18O5/c1-4-13-9(12)7-8(11)10(14-5-2)15-6-3/h10H,4-7H2,1-3H3 | |
Source | PubChem | |
URL | https://pubchem.ncbi.nlm.nih.gov | |
Description | Data deposited in or computed by PubChem | |
InChI Key |
HYVRRAANXHHPGH-UHFFFAOYSA-N | |
Source | PubChem | |
URL | https://pubchem.ncbi.nlm.nih.gov | |
Description | Data deposited in or computed by PubChem | |
Canonical SMILES |
CCOC(C(=O)CC(=O)OCC)OCC | |
Source | PubChem | |
URL | https://pubchem.ncbi.nlm.nih.gov | |
Description | Data deposited in or computed by PubChem | |
Molecular Formula |
C10H18O5 | |
Source | PubChem | |
URL | https://pubchem.ncbi.nlm.nih.gov | |
Description | Data deposited in or computed by PubChem | |
DSSTOX Substance ID |
DTXSID00456624 | |
Record name | Ethyl 4,4-diethoxy-3-oxobutanoate | |
Source | EPA DSSTox | |
URL | https://comptox.epa.gov/dashboard/DTXSID00456624 | |
Description | DSSTox provides a high quality public chemistry resource for supporting improved predictive toxicology. | |
Molecular Weight |
218.25 g/mol | |
Source | PubChem | |
URL | https://pubchem.ncbi.nlm.nih.gov | |
Description | Data deposited in or computed by PubChem | |
Product Name |
Ethyl 4,4-diethoxy-3-oxobutanoate | |
CAS RN |
10495-09-7 | |
Record name | Ethyl 4,4-diethoxy-3-oxobutanoate | |
Source | EPA DSSTox | |
URL | https://comptox.epa.gov/dashboard/DTXSID00456624 | |
Description | DSSTox provides a high quality public chemistry resource for supporting improved predictive toxicology. | |
Record name | ethyl 4,4-diethoxy-3-oxobutanoate | |
Source | European Chemicals Agency (ECHA) | |
URL | https://echa.europa.eu/information-on-chemicals | |
Description | The European Chemicals Agency (ECHA) is an agency of the European Union which is the driving force among regulatory authorities in implementing the EU's groundbreaking chemicals legislation for the benefit of human health and the environment as well as for innovation and competitiveness. | |
Explanation | Use of the information, documents and data from the ECHA website is subject to the terms and conditions of this Legal Notice, and subject to other binding limitations provided for under applicable law, the information, documents and data made available on the ECHA website may be reproduced, distributed and/or used, totally or in part, for non-commercial purposes provided that ECHA is acknowledged as the source: "Source: European Chemicals Agency, http://echa.europa.eu/". Such acknowledgement must be included in each copy of the material. ECHA permits and encourages organisations and individuals to create links to the ECHA website under the following cumulative conditions: Links can only be made to webpages that provide a link to the Legal Notice page. | |
Synthesis routes and methods I
Procedure details
Synthesis routes and methods II
Procedure details
Retrosynthesis Analysis
AI-Powered Synthesis Planning: Our tool employs the Template_relevance Pistachio, Template_relevance Bkms_metabolic, Template_relevance Pistachio_ringbreaker, Template_relevance Reaxys, Template_relevance Reaxys_biocatalysis model, leveraging a vast database of chemical reactions to predict feasible synthetic routes.
One-Step Synthesis Focus: Specifically designed for one-step synthesis, it provides concise and direct routes for your target compounds, streamlining the synthesis process.
Accurate Predictions: Utilizing the extensive PISTACHIO, BKMS_METABOLIC, PISTACHIO_RINGBREAKER, REAXYS, REAXYS_BIOCATALYSIS database, our tool offers high-accuracy predictions, reflecting the latest in chemical research and data.
Strategy Settings
Precursor scoring | Relevance Heuristic |
---|---|
Min. plausibility | 0.01 |
Model | Template_relevance |
Template Set | Pistachio/Bkms_metabolic/Pistachio_ringbreaker/Reaxys/Reaxys_biocatalysis |
Top-N result to add to graph | 6 |
Feasible Synthetic Routes
Q & A
Q1: What is the role of Ethyl 4,4-diethoxy-3-oxobutanoate in the synthesis of 1-(4-ethoxyphenyl)-5-formyl-1H-1,2,3-triazole-4-carboxylic acid?
A1: Ethyl 4,4-diethoxy-3-oxobutanoate serves as a crucial building block in the synthesis of 1-(4-ethoxyphenyl)-5-formyl-1H-1,2,3-triazole-4-carboxylic acid []. It reacts with 1-azido-4-ethoxybenzene under basic conditions to form ethyl 5-(diethoxymethyl)-1-(4-ethoxyphenyl)-1H-1,2,3-triazole-4-carboxylate. This intermediate contains protected formyl and acid groups. Subsequent reactions then yield the target compound, 1-(4-ethoxyphenyl)-5-formyl-1H-1,2,3-triazole-4-carboxylic acid.
Q2: Why is Ethyl 4,4-diethoxy-3-oxobutanoate used with protected functional groups in this synthesis?
A2: The use of Ethyl 4,4-diethoxy-3-oxobutanoate with protected formyl and acid groups allows for greater control over the reaction pathway []. By masking these reactive groups, unwanted side reactions can be minimized, leading to a more efficient and selective synthesis of the desired 1,2,3-triazole derivative. This approach highlights the importance of protecting group strategies in organic synthesis.
Disclaimer and Information on In-Vitro Research Products
Please be aware that all articles and product information presented on BenchChem are intended solely for informational purposes. The products available for purchase on BenchChem are specifically designed for in-vitro studies, which are conducted outside of living organisms. In-vitro studies, derived from the Latin term "in glass," involve experiments performed in controlled laboratory settings using cells or tissues. It is important to note that these products are not categorized as medicines or drugs, and they have not received approval from the FDA for the prevention, treatment, or cure of any medical condition, ailment, or disease. We must emphasize that any form of bodily introduction of these products into humans or animals is strictly prohibited by law. It is essential to adhere to these guidelines to ensure compliance with legal and ethical standards in research and experimentation.