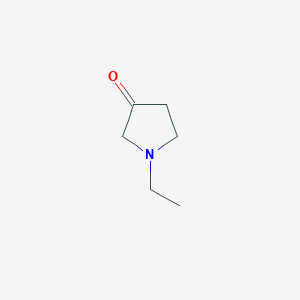
1-Ethylpyrrolidin-3-one
- Click on QUICK INQUIRY to receive a quote from our team of experts.
- With the quality product at a COMPETITIVE price, you can focus more on your research.
Overview
Description
1-Ethylpyrrolidin-3-one is a five-membered nitrogen-containing heterocyclic compound. It is a derivative of pyrrolidinone, where an ethyl group is attached to the nitrogen atom. This compound is of significant interest due to its versatile applications in various fields, including medicinal chemistry and industrial processes.
Preparation Methods
1-Ethylpyrrolidin-3-one can be synthesized through several methods. One common synthetic route involves the reaction of 3-pyrrolidinone with ethyl iodide in the presence of a base such as potassium carbonate. The reaction typically occurs under reflux conditions, leading to the formation of this compound.
Industrial production methods often involve the catalytic hydrogenation of 1-ethyl-2-pyrrolidinone. This process uses a metal catalyst, such as palladium on carbon, under high pressure and temperature to achieve the desired product.
Chemical Reactions Analysis
1-Ethylpyrrolidin-3-one undergoes various chemical reactions, including:
Oxidation: It can be oxidized to form corresponding lactams or other oxidized derivatives.
Reduction: Reduction reactions can convert it into different amines or alcohols.
Substitution: Nucleophilic substitution reactions can introduce various functional groups into the molecule.
Common reagents used in these reactions include oxidizing agents like potassium permanganate, reducing agents like lithium aluminum hydride, and nucleophiles such as alkyl halides. The major products formed depend on the specific reaction conditions and reagents used.
Scientific Research Applications
1-Ethylpyrrolidin-3-one has a wide range of applications in scientific research:
Chemistry: It serves as a building block for synthesizing more complex molecules and as a solvent in various chemical reactions.
Biology: It is used in the synthesis of biologically active compounds, including pharmaceuticals and agrochemicals.
Medicine: Derivatives of this compound are investigated for their potential therapeutic properties, such as antimicrobial and anticancer activities.
Industry: It is used in the production of polymers, resins, and other industrial chemicals.
Mechanism of Action
The mechanism of action of 1-ethylpyrrolidin-3-one and its derivatives often involves interaction with specific molecular targets, such as enzymes or receptors. These interactions can modulate biological pathways, leading to the desired therapeutic effects. For example, some derivatives may inhibit enzyme activity, thereby preventing the progression of certain diseases.
Comparison with Similar Compounds
1-Ethylpyrrolidin-3-one can be compared with other similar compounds, such as:
Pyrrolidin-2-one: Lacks the ethyl group, leading to different chemical and biological properties.
1-Methylpyrrolidin-3-one: Similar structure but with a methyl group instead of an ethyl group, affecting its reactivity and applications.
Pyrrolidine: The parent compound without the carbonyl group, resulting in different chemical behavior.
The uniqueness of this compound lies in its specific substitution pattern, which imparts distinct chemical and biological properties, making it valuable for various applications.
Biological Activity
1-Ethylpyrrolidin-3-one, also known as 1-Ethyl-3-pyrrolidinone (EP), is a cyclic amine that has garnered attention for its diverse biological activities. This compound is primarily studied for its potential applications in medicinal chemistry, including antimicrobial and antifungal properties, as well as its role in drug discovery.
This compound is characterized by its unique pyrrolidine structure, which contributes to its reactivity and interaction with biological systems. The compound can act as a nucleophile , engaging in substitution reactions with electrophilic centers in other molecules. This ability allows it to modulate the activity of various enzymes and receptors, influencing physiological responses in biological systems .
Antimicrobial and Antifungal Properties
Research indicates that this compound exhibits significant antimicrobial and antifungal activities. In a study evaluating the antimicrobial efficacy of pyrrolidine derivatives, this compound showed promising results against a range of pathogens, suggesting its potential as a lead compound in the development of new antimicrobial agents .
Structure-Activity Relationship (SAR)
The biological activity of this compound can be influenced by structural modifications. Variations in the substituents on the pyrrolidine ring have been shown to affect the compound's potency and selectivity against specific biological targets. For instance, studies have demonstrated that certain derivatives possess enhanced activity against specific bacterial strains or fungi, highlighting the importance of SAR in optimizing therapeutic efficacy .
Case Studies
Case Study 1: Antimicrobial Efficacy
In a controlled experiment, this compound was tested against common bacterial strains including Staphylococcus aureus and Escherichia coli. The results indicated an inhibition zone diameter of 15 mm against S. aureus, demonstrating moderate antibacterial activity. The compound's effectiveness was further enhanced when combined with other known antibiotics, suggesting potential for use in combination therapies.
Case Study 2: Antifungal Activity
Another study assessed the antifungal properties of this compound against Candida albicans. The compound exhibited a minimum inhibitory concentration (MIC) of 32 µg/mL, indicating significant antifungal activity. This finding supports the notion that pyrrolidine derivatives can be developed into effective antifungal agents .
Applications in Drug Discovery
The versatility of this compound extends to its application in drug discovery. Its structure serves as a scaffold for synthesizing novel bioactive compounds. Research has shown that modifications to the ethyl group or the nitrogen atom can lead to derivatives with improved pharmacological profiles, including enhanced bioavailability and reduced toxicity .
Summary Table of Biological Activities
Properties
IUPAC Name |
1-ethylpyrrolidin-3-one |
Source
|
---|---|---|
Source | PubChem | |
URL | https://pubchem.ncbi.nlm.nih.gov | |
Description | Data deposited in or computed by PubChem | |
InChI |
InChI=1S/C6H11NO/c1-2-7-4-3-6(8)5-7/h2-5H2,1H3 |
Source
|
Source | PubChem | |
URL | https://pubchem.ncbi.nlm.nih.gov | |
Description | Data deposited in or computed by PubChem | |
InChI Key |
XXXQFHALDVELNQ-UHFFFAOYSA-N |
Source
|
Source | PubChem | |
URL | https://pubchem.ncbi.nlm.nih.gov | |
Description | Data deposited in or computed by PubChem | |
Canonical SMILES |
CCN1CCC(=O)C1 |
Source
|
Source | PubChem | |
URL | https://pubchem.ncbi.nlm.nih.gov | |
Description | Data deposited in or computed by PubChem | |
Molecular Formula |
C6H11NO |
Source
|
Source | PubChem | |
URL | https://pubchem.ncbi.nlm.nih.gov | |
Description | Data deposited in or computed by PubChem | |
DSSTOX Substance ID |
DTXSID80317582 |
Source
|
Record name | 1-ethylpyrrolidin-3-one | |
Source | EPA DSSTox | |
URL | https://comptox.epa.gov/dashboard/DTXSID80317582 | |
Description | DSSTox provides a high quality public chemistry resource for supporting improved predictive toxicology. | |
Molecular Weight |
113.16 g/mol |
Source
|
Source | PubChem | |
URL | https://pubchem.ncbi.nlm.nih.gov | |
Description | Data deposited in or computed by PubChem | |
CAS No. |
102153-86-6 |
Source
|
Record name | 1-ethylpyrrolidin-3-one | |
Source | EPA DSSTox | |
URL | https://comptox.epa.gov/dashboard/DTXSID80317582 | |
Description | DSSTox provides a high quality public chemistry resource for supporting improved predictive toxicology. | |
Disclaimer and Information on In-Vitro Research Products
Please be aware that all articles and product information presented on BenchChem are intended solely for informational purposes. The products available for purchase on BenchChem are specifically designed for in-vitro studies, which are conducted outside of living organisms. In-vitro studies, derived from the Latin term "in glass," involve experiments performed in controlled laboratory settings using cells or tissues. It is important to note that these products are not categorized as medicines or drugs, and they have not received approval from the FDA for the prevention, treatment, or cure of any medical condition, ailment, or disease. We must emphasize that any form of bodily introduction of these products into humans or animals is strictly prohibited by law. It is essential to adhere to these guidelines to ensure compliance with legal and ethical standards in research and experimentation.