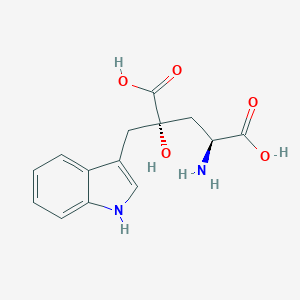
Monatin
Overview
Description
Preparation Methods
Synthetic Routes and Reaction Conditions: Monatin can be synthesized through a series of reactions starting from commercially available indole acetic acid. The synthetic route involves a cross-aldol reaction between pyruvic acid and indole-3-pyruvic acid to form the key intermediate, 4-hydroxy-4-(3-indolylmethyl)-2-ketoglutaric acid. This intermediate undergoes subsequent reactions, including conversion to a hydroxyimino group with hydroxylamine and then to an amino group via hydrogenation, to produce this compound .
Industrial Production Methods: Industrial production of this compound involves the isolation of the compound from the plant Sclerochiton ilicifolius. The process includes extraction, purification, and crystallization steps to obtain this compound in its pure form. The production methods aim to maximize yield and purity while maintaining the natural sweetness properties of the compound .
Chemical Reactions Analysis
Types of Reactions: Monatin undergoes various chemical reactions, including:
Oxidation: this compound can be oxidized to form corresponding oxo derivatives.
Reduction: Reduction reactions can convert this compound to its reduced forms.
Substitution: this compound can undergo substitution reactions, particularly at the amino and carboxylic acid functional groups.
Common Reagents and Conditions:
Oxidation: Common oxidizing agents include potassium permanganate and hydrogen peroxide.
Reduction: Reducing agents such as sodium borohydride and lithium aluminum hydride are used.
Substitution: Reagents like acyl chlorides and alkyl halides are commonly employed.
Major Products: The major products formed from these reactions include various derivatives of this compound, such as oxo-monatin, reduced this compound, and substituted this compound derivatives .
Scientific Research Applications
Monatin has a wide range of scientific research applications, including:
Chemistry: this compound is studied for its unique sweetening properties and potential as a natural sweetener in food and beverage industries.
Biology: Research focuses on the metabolic pathways and biosynthesis of this compound in plants.
Medicine: this compound’s potential health benefits, such as its low-calorie content and suitability for diabetic patients, are being explored.
Industry: this compound is used in the formulation of low-calorie and sugar-free products, providing a natural alternative to artificial sweeteners
Mechanism of Action
Monatin exerts its sweetening effect by interacting with the sweet taste receptors on the tongue. The compound binds to the T1R2 and T1R3 receptor subunits, which are part of the G-protein-coupled receptor family. This binding triggers a signal transduction pathway that results in the perception of sweetness. The specific molecular interactions and pathways involved in this process are still under investigation .
Comparison with Similar Compounds
Monatin is unique among sweeteners due to its high sweetness intensity and natural origin. Similar compounds include:
Aspartame: An artificial sweetener that is about 200 times sweeter than sucrose.
Steviol glycosides: Natural sweeteners derived from the Stevia plant, with sweetness levels ranging from 50 to 300 times that of sucrose.
Thaumatin: A protein sweetener that is approximately 2000 times sweeter than sucrose.
This compound stands out due to its intense sweetness, natural occurrence, and potential health benefits, making it a promising candidate for various applications in the food and beverage industry .
Properties
IUPAC Name |
(2S,4S)-4-amino-2-hydroxy-2-(1H-indol-3-ylmethyl)pentanedioic acid | |
---|---|---|
Source | PubChem | |
URL | https://pubchem.ncbi.nlm.nih.gov | |
Description | Data deposited in or computed by PubChem | |
InChI |
InChI=1S/C14H16N2O5/c15-10(12(17)18)6-14(21,13(19)20)5-8-7-16-11-4-2-1-3-9(8)11/h1-4,7,10,16,21H,5-6,15H2,(H,17,18)(H,19,20)/t10-,14-/m0/s1 | |
Source | PubChem | |
URL | https://pubchem.ncbi.nlm.nih.gov | |
Description | Data deposited in or computed by PubChem | |
InChI Key |
RMLYXMMBIZLGAQ-HZMBPMFUSA-N | |
Source | PubChem | |
URL | https://pubchem.ncbi.nlm.nih.gov | |
Description | Data deposited in or computed by PubChem | |
Canonical SMILES |
C1=CC=C2C(=C1)C(=CN2)CC(CC(C(=O)O)N)(C(=O)O)O | |
Source | PubChem | |
URL | https://pubchem.ncbi.nlm.nih.gov | |
Description | Data deposited in or computed by PubChem | |
Isomeric SMILES |
C1=CC=C2C(=C1)C(=CN2)C[C@](C[C@@H](C(=O)O)N)(C(=O)O)O | |
Source | PubChem | |
URL | https://pubchem.ncbi.nlm.nih.gov | |
Description | Data deposited in or computed by PubChem | |
Molecular Formula |
C14H16N2O5 | |
Source | PubChem | |
URL | https://pubchem.ncbi.nlm.nih.gov | |
Description | Data deposited in or computed by PubChem | |
DSSTOX Substance ID |
DTXSID60432020 | |
Record name | Monatin | |
Source | EPA DSSTox | |
URL | https://comptox.epa.gov/dashboard/DTXSID60432020 | |
Description | DSSTox provides a high quality public chemistry resource for supporting improved predictive toxicology. | |
Molecular Weight |
292.29 g/mol | |
Source | PubChem | |
URL | https://pubchem.ncbi.nlm.nih.gov | |
Description | Data deposited in or computed by PubChem | |
CAS No. |
146142-94-1 | |
Record name | Monatin | |
Source | CAS Common Chemistry | |
URL | https://commonchemistry.cas.org/detail?cas_rn=146142-94-1 | |
Description | CAS Common Chemistry is an open community resource for accessing chemical information. Nearly 500,000 chemical substances from CAS REGISTRY cover areas of community interest, including common and frequently regulated chemicals, and those relevant to high school and undergraduate chemistry classes. This chemical information, curated by our expert scientists, is provided in alignment with our mission as a division of the American Chemical Society. | |
Explanation | The data from CAS Common Chemistry is provided under a CC-BY-NC 4.0 license, unless otherwise stated. | |
Record name | Monatin | |
Source | EPA DSSTox | |
URL | https://comptox.epa.gov/dashboard/DTXSID60432020 | |
Description | DSSTox provides a high quality public chemistry resource for supporting improved predictive toxicology. | |
Record name | Monatin, (2S,4S)- | |
Source | FDA Global Substance Registration System (GSRS) | |
URL | https://gsrs.ncats.nih.gov/ginas/app/beta/substances/TL342VQ3GR | |
Description | The FDA Global Substance Registration System (GSRS) enables the efficient and accurate exchange of information on what substances are in regulated products. Instead of relying on names, which vary across regulatory domains, countries, and regions, the GSRS knowledge base makes it possible for substances to be defined by standardized, scientific descriptions. | |
Explanation | Unless otherwise noted, the contents of the FDA website (www.fda.gov), both text and graphics, are not copyrighted. They are in the public domain and may be republished, reprinted and otherwise used freely by anyone without the need to obtain permission from FDA. Credit to the U.S. Food and Drug Administration as the source is appreciated but not required. | |
Synthesis routes and methods I
Procedure details
Synthesis routes and methods II
Procedure details
Retrosynthesis Analysis
AI-Powered Synthesis Planning: Our tool employs the Template_relevance Pistachio, Template_relevance Bkms_metabolic, Template_relevance Pistachio_ringbreaker, Template_relevance Reaxys, Template_relevance Reaxys_biocatalysis model, leveraging a vast database of chemical reactions to predict feasible synthetic routes.
One-Step Synthesis Focus: Specifically designed for one-step synthesis, it provides concise and direct routes for your target compounds, streamlining the synthesis process.
Accurate Predictions: Utilizing the extensive PISTACHIO, BKMS_METABOLIC, PISTACHIO_RINGBREAKER, REAXYS, REAXYS_BIOCATALYSIS database, our tool offers high-accuracy predictions, reflecting the latest in chemical research and data.
Strategy Settings
Precursor scoring | Relevance Heuristic |
---|---|
Min. plausibility | 0.01 |
Model | Template_relevance |
Template Set | Pistachio/Bkms_metabolic/Pistachio_ringbreaker/Reaxys/Reaxys_biocatalysis |
Top-N result to add to graph | 6 |
Feasible Synthetic Routes
Disclaimer and Information on In-Vitro Research Products
Please be aware that all articles and product information presented on BenchChem are intended solely for informational purposes. The products available for purchase on BenchChem are specifically designed for in-vitro studies, which are conducted outside of living organisms. In-vitro studies, derived from the Latin term "in glass," involve experiments performed in controlled laboratory settings using cells or tissues. It is important to note that these products are not categorized as medicines or drugs, and they have not received approval from the FDA for the prevention, treatment, or cure of any medical condition, ailment, or disease. We must emphasize that any form of bodily introduction of these products into humans or animals is strictly prohibited by law. It is essential to adhere to these guidelines to ensure compliance with legal and ethical standards in research and experimentation.