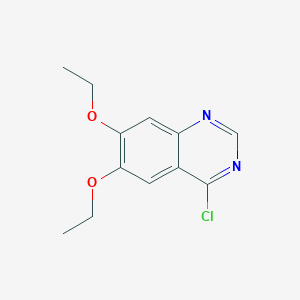
4-Chloro-6,7-diethoxyquinazoline
Overview
Description
4-Chloro-6,7-diethoxyquinazoline is a heterocyclic organic compound belonging to the quinazoline family. This compound has garnered significant attention in the scientific community due to its potential therapeutic and industrial applications. Its molecular formula is C12H13ClN2O2, and it is characterized by the presence of a quinazoline ring substituted with chlorine and ethoxy groups.
Mechanism of Action
Target of Action
4-Chloro-6,7-diethoxyquinazoline is a novel compound that has been evaluated for its antiproliferative activities against human cancer cell lines . The primary targets of this compound are cancer cells, particularly MGC-803 cells . These cells are significantly affected by the compound, which displays potent anti-proliferative activity .
Mode of Action
The compound interacts with its targets by inhibiting the colony formation and migration of MGC-803 cells . This interaction results in significant changes in the cells, including the induction of apoptosis and cell cycle arrest at the G1-phase . This means that the compound prevents the cells from dividing and proliferating, thereby inhibiting the growth of the cancer.
Pharmacokinetics
The compound’s potent anti-proliferative activity against mgc-803 cells suggests that it may have good bioavailability
Result of Action
The result of this compound’s action is a significant reduction in the proliferation of MGC-803 cells . By inducing apoptosis and causing cell cycle arrest, the compound effectively inhibits the growth of these cancer cells . This could potentially lead to a decrease in tumor size and progression.
Biochemical Analysis
Biochemical Properties
The nature of these interactions often involves binding to active sites or allosteric sites, leading to changes in the function of the target molecule .
Cellular Effects
Related quinazoline derivatives have been shown to influence cell function, including impacts on cell signaling pathways, gene expression, and cellular metabolism . These effects can vary depending on the specific cells and the concentration of the compound.
Molecular Mechanism
Quinazoline derivatives often exert their effects at the molecular level through binding interactions with biomolecules, enzyme inhibition or activation, and changes in gene expression .
Preparation Methods
The synthesis of 4-Chloro-6,7-diethoxyquinazoline typically involves several steps:
Nitrification: Starting with a suitable precursor, such as 3,4-diethoxyacetophenone, nitrification is carried out to introduce nitro groups.
Condensation: The nitro compound undergoes condensation with N,N-dimethylformamide dimethyl acetal to form an intermediate.
Reduction Cyclization: The intermediate is then subjected to hydrogenation, leading to reduction and cyclization to form the quinazoline ring.
Chlorination: Finally, chlorination of the quinazoline derivative yields this compound.
These steps are carried out under controlled conditions to ensure high yield and purity. Industrial production methods may involve optimization of these steps to scale up the synthesis while maintaining efficiency and cost-effectiveness.
Chemical Reactions Analysis
4-Chloro-6,7-diethoxyquinazoline undergoes various chemical reactions, including:
Substitution Reactions: The chlorine atom can be substituted with other nucleophiles under appropriate conditions.
Oxidation and Reduction: The compound can be oxidized or reduced to form different derivatives, depending on the reagents and conditions used.
Coupling Reactions: It can participate in coupling reactions with arylamines or phenolic compounds to form azo dyes.
Common reagents used in these reactions include halogenating agents, reducing agents like hydrogen gas, and various nucleophiles. The major products formed depend on the specific reaction conditions and reagents used.
Scientific Research Applications
4-Chloro-6,7-diethoxyquinazoline has a wide range of applications in scientific research:
Chemistry: It serves as a valuable intermediate in the synthesis of various heterocyclic compounds.
Biology: The compound is used in the study of biological pathways and molecular interactions.
Medicine: It has potential therapeutic applications, particularly in the development of anti-tumor agents.
Industry: The compound is used in the production of dyes and other industrial chemicals.
Comparison with Similar Compounds
4-Chloro-6,7-diethoxyquinazoline can be compared with other quinazoline derivatives such as:
4-Chloro-6,7-dimethoxyquinazoline: Similar in structure but with methoxy groups instead of ethoxy groups.
4-Amino-2-chloro-6,7-dimethoxyquinazoline: Contains an amino group, making it useful in the synthesis of azo dyes.
Gefitinib and Afatinib: These are clinically approved anti-cancer drugs containing quinazoline scaffolds.
The uniqueness of this compound lies in its specific substitutions, which confer distinct chemical properties and biological activities.
Properties
IUPAC Name |
4-chloro-6,7-diethoxyquinazoline | |
---|---|---|
Source | PubChem | |
URL | https://pubchem.ncbi.nlm.nih.gov | |
Description | Data deposited in or computed by PubChem | |
InChI |
InChI=1S/C12H13ClN2O2/c1-3-16-10-5-8-9(6-11(10)17-4-2)14-7-15-12(8)13/h5-7H,3-4H2,1-2H3 | |
Source | PubChem | |
URL | https://pubchem.ncbi.nlm.nih.gov | |
Description | Data deposited in or computed by PubChem | |
InChI Key |
HGMQPDNSSYTATF-UHFFFAOYSA-N | |
Source | PubChem | |
URL | https://pubchem.ncbi.nlm.nih.gov | |
Description | Data deposited in or computed by PubChem | |
Canonical SMILES |
CCOC1=C(C=C2C(=C1)C(=NC=N2)Cl)OCC | |
Source | PubChem | |
URL | https://pubchem.ncbi.nlm.nih.gov | |
Description | Data deposited in or computed by PubChem | |
Molecular Formula |
C12H13ClN2O2 | |
Source | PubChem | |
URL | https://pubchem.ncbi.nlm.nih.gov | |
Description | Data deposited in or computed by PubChem | |
DSSTOX Substance ID |
DTXSID50470633 | |
Record name | 4-Chloro-6,7-diethoxyquinazoline | |
Source | EPA DSSTox | |
URL | https://comptox.epa.gov/dashboard/DTXSID50470633 | |
Description | DSSTox provides a high quality public chemistry resource for supporting improved predictive toxicology. | |
Molecular Weight |
252.69 g/mol | |
Source | PubChem | |
URL | https://pubchem.ncbi.nlm.nih.gov | |
Description | Data deposited in or computed by PubChem | |
CAS No. |
162363-46-4 | |
Record name | 4-Chloro-6,7-diethoxyquinazoline | |
Source | EPA DSSTox | |
URL | https://comptox.epa.gov/dashboard/DTXSID50470633 | |
Description | DSSTox provides a high quality public chemistry resource for supporting improved predictive toxicology. | |
Synthesis routes and methods
Procedure details
Retrosynthesis Analysis
AI-Powered Synthesis Planning: Our tool employs the Template_relevance Pistachio, Template_relevance Bkms_metabolic, Template_relevance Pistachio_ringbreaker, Template_relevance Reaxys, Template_relevance Reaxys_biocatalysis model, leveraging a vast database of chemical reactions to predict feasible synthetic routes.
One-Step Synthesis Focus: Specifically designed for one-step synthesis, it provides concise and direct routes for your target compounds, streamlining the synthesis process.
Accurate Predictions: Utilizing the extensive PISTACHIO, BKMS_METABOLIC, PISTACHIO_RINGBREAKER, REAXYS, REAXYS_BIOCATALYSIS database, our tool offers high-accuracy predictions, reflecting the latest in chemical research and data.
Strategy Settings
Precursor scoring | Relevance Heuristic |
---|---|
Min. plausibility | 0.01 |
Model | Template_relevance |
Template Set | Pistachio/Bkms_metabolic/Pistachio_ringbreaker/Reaxys/Reaxys_biocatalysis |
Top-N result to add to graph | 6 |
Feasible Synthetic Routes
Disclaimer and Information on In-Vitro Research Products
Please be aware that all articles and product information presented on BenchChem are intended solely for informational purposes. The products available for purchase on BenchChem are specifically designed for in-vitro studies, which are conducted outside of living organisms. In-vitro studies, derived from the Latin term "in glass," involve experiments performed in controlled laboratory settings using cells or tissues. It is important to note that these products are not categorized as medicines or drugs, and they have not received approval from the FDA for the prevention, treatment, or cure of any medical condition, ailment, or disease. We must emphasize that any form of bodily introduction of these products into humans or animals is strictly prohibited by law. It is essential to adhere to these guidelines to ensure compliance with legal and ethical standards in research and experimentation.