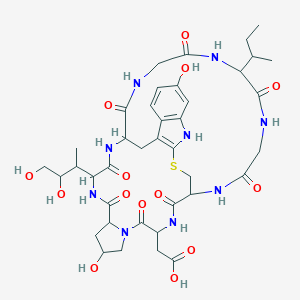
Amanitins
Overview
Description
Amanitins are a group of highly toxic bicyclic octapeptides found in several species of the Amanita genus, most notably in Amanita phalloides (death cap mushroom), Amanita virosa (destroying angel), and Amanita verna . These compounds are known for their potent inhibition of RNA polymerase II, leading to severe and often fatal poisoning in humans and animals .
Preparation Methods
Synthetic Routes and Reaction Conditions: The synthesis of amanitins is complex due to their intricate bicyclic structure. The process involves multiple steps, including peptide bond formation, cyclization, and oxidation. The key steps include:
Peptide Bond Formation: This is typically achieved using solid-phase peptide synthesis (SPPS) techniques.
Cyclization: The linear peptide is cyclized to form the bicyclic structure.
Oxidation: Specific amino acid residues are oxidized to achieve the final structure.
Industrial Production Methods: Industrial production of this compound is not common due to their high toxicity and complex synthesis. Instead, these compounds are usually extracted from Amanita mushrooms using methods such as solvent extraction, column chromatography, and high-performance liquid chromatography (HPLC) .
Chemical Reactions Analysis
Types of Reactions: Amanitins undergo various chemical reactions, including:
Oxidation: Specific residues within the peptide can be oxidized to form the active structure.
Reduction: Reduction reactions can reverse the oxidation state of certain residues.
Substitution: Amino acid residues can be substituted to create analogs with different properties.
Common Reagents and Conditions:
Oxidation: Hydrogen peroxide or other oxidizing agents under controlled conditions.
Reduction: Reducing agents such as dithiothreitol (DTT).
Substitution: Amino acid derivatives and coupling reagents used in peptide synthesis.
Major Products Formed: The major products formed from these reactions are various analogs of this compound, which can have different biological activities and toxicities .
Scientific Research Applications
Amanitins have several scientific research applications, including:
Chemistry: Used as tools to study peptide synthesis and structure-activity relationships.
Biology: Employed to investigate the mechanisms of RNA polymerase II inhibition and transcription regulation.
Industry: Utilized in the development of antibody-drug conjugates (ADCs) for targeted cancer therapy.
Mechanism of Action
Amanitins exert their effects by binding to and inhibiting RNA polymerase II, an enzyme crucial for the transcription of DNA into messenger RNA (mRNA). This inhibition prevents the synthesis of mRNA, leading to a halt in protein production and ultimately causing cell death. The primary molecular targets are the largest subunit of RNA polymerase II, RNApb1, bridge helix, and trigger loop .
Comparison with Similar Compounds
Phallotoxins: Another group of toxins found in Amanita mushrooms, which primarily target actin filaments in cells.
Virotoxins: Similar to phallotoxins but with different structural features and targets.
Uniqueness of Amanitins: this compound are unique due to their specific inhibition of RNA polymerase II, which is not observed with phallotoxins or virotoxins. This unique mechanism makes this compound particularly potent and deadly .
Properties
IUPAC Name |
2-[34-butan-2-yl-13-(3,4-dihydroxybutan-2-yl)-8,22-dihydroxy-2,5,11,14,30,33,36,39-octaoxo-27-thia-3,6,12,15,25,29,32,35,38-nonazapentacyclo[14.12.11.06,10.018,26.019,24]nonatriaconta-18(26),19(24),20,22-tetraen-4-yl]acetic acid | |
---|---|---|
Source | PubChem | |
URL | https://pubchem.ncbi.nlm.nih.gov | |
Description | Data deposited in or computed by PubChem | |
InChI |
InChI=1S/C39H53N9O14S/c1-4-16(2)31-36(60)41-11-28(53)42-25-15-63-38-21(20-6-5-18(50)7-22(20)45-38)9-23(33(57)40-12-29(54)46-31)43-37(61)32(17(3)27(52)14-49)47-35(59)26-8-19(51)13-48(26)39(62)24(10-30(55)56)44-34(25)58/h5-7,16-17,19,23-27,31-32,45,49-52H,4,8-15H2,1-3H3,(H,40,57)(H,41,60)(H,42,53)(H,43,61)(H,44,58)(H,46,54)(H,47,59)(H,55,56) | |
Source | PubChem | |
URL | https://pubchem.ncbi.nlm.nih.gov | |
Description | Data deposited in or computed by PubChem | |
InChI Key |
RUDNHCHNENLLKM-UHFFFAOYSA-N | |
Source | PubChem | |
URL | https://pubchem.ncbi.nlm.nih.gov | |
Description | Data deposited in or computed by PubChem | |
Canonical SMILES |
CCC(C)C1C(=O)NCC(=O)NC2CSC3=C(CC(C(=O)NCC(=O)N1)NC(=O)C(NC(=O)C4CC(CN4C(=O)C(NC2=O)CC(=O)O)O)C(C)C(CO)O)C5=C(N3)C=C(C=C5)O | |
Source | PubChem | |
URL | https://pubchem.ncbi.nlm.nih.gov | |
Description | Data deposited in or computed by PubChem | |
Molecular Formula |
C39H53N9O14S | |
Source | PubChem | |
URL | https://pubchem.ncbi.nlm.nih.gov | |
Description | Data deposited in or computed by PubChem | |
Molecular Weight |
904.0 g/mol | |
Source | PubChem | |
URL | https://pubchem.ncbi.nlm.nih.gov | |
Description | Data deposited in or computed by PubChem | |
CAS No. |
11030-71-0 | |
Record name | Amanitin | |
Source | ChemIDplus | |
URL | https://pubchem.ncbi.nlm.nih.gov/substance/?source=chemidplus&sourceid=0011030710 | |
Description | ChemIDplus is a free, web search system that provides access to the structure and nomenclature authority files used for the identification of chemical substances cited in National Library of Medicine (NLM) databases, including the TOXNET system. | |
Retrosynthesis Analysis
AI-Powered Synthesis Planning: Our tool employs the Template_relevance Pistachio, Template_relevance Bkms_metabolic, Template_relevance Pistachio_ringbreaker, Template_relevance Reaxys, Template_relevance Reaxys_biocatalysis model, leveraging a vast database of chemical reactions to predict feasible synthetic routes.
One-Step Synthesis Focus: Specifically designed for one-step synthesis, it provides concise and direct routes for your target compounds, streamlining the synthesis process.
Accurate Predictions: Utilizing the extensive PISTACHIO, BKMS_METABOLIC, PISTACHIO_RINGBREAKER, REAXYS, REAXYS_BIOCATALYSIS database, our tool offers high-accuracy predictions, reflecting the latest in chemical research and data.
Strategy Settings
Precursor scoring | Relevance Heuristic |
---|---|
Min. plausibility | 0.01 |
Model | Template_relevance |
Template Set | Pistachio/Bkms_metabolic/Pistachio_ringbreaker/Reaxys/Reaxys_biocatalysis |
Top-N result to add to graph | 6 |
Feasible Synthetic Routes
Disclaimer and Information on In-Vitro Research Products
Please be aware that all articles and product information presented on BenchChem are intended solely for informational purposes. The products available for purchase on BenchChem are specifically designed for in-vitro studies, which are conducted outside of living organisms. In-vitro studies, derived from the Latin term "in glass," involve experiments performed in controlled laboratory settings using cells or tissues. It is important to note that these products are not categorized as medicines or drugs, and they have not received approval from the FDA for the prevention, treatment, or cure of any medical condition, ailment, or disease. We must emphasize that any form of bodily introduction of these products into humans or animals is strictly prohibited by law. It is essential to adhere to these guidelines to ensure compliance with legal and ethical standards in research and experimentation.