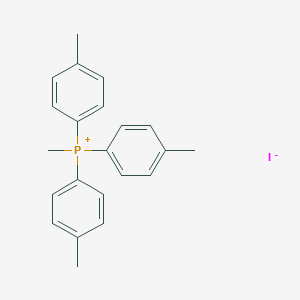
Methyltris(4-methylphenyl)phosphonium iodide
- Click on QUICK INQUIRY to receive a quote from our team of experts.
- With the quality product at a COMPETITIVE price, you can focus more on your research.
Overview
Description
Safety and Hazards
The safety data sheet for a similar compound, Methyltriphenylphosphonium iodide, indicates that it is considered hazardous by the 2012 OSHA Hazard Communication Standard . It is toxic if swallowed and may cause respiratory irritation . It is recommended to avoid breathing dust/fume/gas/mist/vapors/spray and to use only outdoors or in a well-ventilated area .
Future Directions
While the specific future directions for “Methyltris(4-methylphenyl)phosphonium iodide” are not mentioned in the retrieved data, the field of photoredox catalysis, in which such compounds play a crucial role, has seen numerous remarkable breakthroughs and notable progress over the past few decades . The use of cost-effective and easily accessible iodide/phosphine catalyst systems offers a more sustainable and economically viable approach . This suggests that the study and application of “Methyltris(4-methylphenyl)phosphonium iodide” and similar compounds may continue to be an area of interest in the future.
properties
IUPAC Name |
methyl-tris(4-methylphenyl)phosphanium;iodide |
Source
|
---|---|---|
Source | PubChem | |
URL | https://pubchem.ncbi.nlm.nih.gov | |
Description | Data deposited in or computed by PubChem | |
InChI |
InChI=1S/C22H24P.HI/c1-17-5-11-20(12-6-17)23(4,21-13-7-18(2)8-14-21)22-15-9-19(3)10-16-22;/h5-16H,1-4H3;1H/q+1;/p-1 |
Source
|
Source | PubChem | |
URL | https://pubchem.ncbi.nlm.nih.gov | |
Description | Data deposited in or computed by PubChem | |
InChI Key |
SUQPXWWSBRNXEE-UHFFFAOYSA-M |
Source
|
Source | PubChem | |
URL | https://pubchem.ncbi.nlm.nih.gov | |
Description | Data deposited in or computed by PubChem | |
Canonical SMILES |
CC1=CC=C(C=C1)[P+](C)(C2=CC=C(C=C2)C)C3=CC=C(C=C3)C.[I-] |
Source
|
Source | PubChem | |
URL | https://pubchem.ncbi.nlm.nih.gov | |
Description | Data deposited in or computed by PubChem | |
Molecular Formula |
C22H24IP |
Source
|
Source | PubChem | |
URL | https://pubchem.ncbi.nlm.nih.gov | |
Description | Data deposited in or computed by PubChem | |
Molecular Weight |
446.3 g/mol |
Source
|
Source | PubChem | |
URL | https://pubchem.ncbi.nlm.nih.gov | |
Description | Data deposited in or computed by PubChem | |
Synthesis routes and methods
Procedure details
Disclaimer and Information on In-Vitro Research Products
Please be aware that all articles and product information presented on BenchChem are intended solely for informational purposes. The products available for purchase on BenchChem are specifically designed for in-vitro studies, which are conducted outside of living organisms. In-vitro studies, derived from the Latin term "in glass," involve experiments performed in controlled laboratory settings using cells or tissues. It is important to note that these products are not categorized as medicines or drugs, and they have not received approval from the FDA for the prevention, treatment, or cure of any medical condition, ailment, or disease. We must emphasize that any form of bodily introduction of these products into humans or animals is strictly prohibited by law. It is essential to adhere to these guidelines to ensure compliance with legal and ethical standards in research and experimentation.