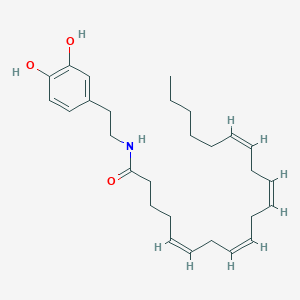
N-Arachidonyldopamine
Overview
Description
N-Arachidonoyl dopamine (NADA) is an intriguing endocannabinoid that plays dual roles as an agonist for the CB₁ receptor and the transient receptor potential V₁ (TRPV1) ion channel . First described as a putative endocannabinoid in 2000, NADA was later identified as an endovanilloid in 2002. It is an endogenous lipid derived from arachidonic acid and dopamine, with particularly high concentrations in brain regions like the hippocampus, cerebellum, and striatum .
Preparation Methods
Synthetic Routes:: NADA can be synthesized through enzymatic pathways involving arachidonic acid and dopamine. specific synthetic routes are not widely documented.
Industrial Production:: Industrial-scale production methods for NADA remain limited due to its complex structure and low abundance. Research efforts are ongoing to explore scalable synthesis routes.
Chemical Reactions Analysis
Table 1: Biosynthetic Mechanisms of NADA
Critical Notes :
-
NA-tyrosine, identified endogenously, does not act as a precursor .
-
FAAH knockout models show reduced striatal NADA levels, confirming its regulatory role .
Metabolic Degradation
NADA undergoes enzymatic hydrolysis and methylation:
Table 2: Metabolic Pathways of NADA
Key Observations :
-
COMT-mediated methylation inactivates NADA’s TRPV1 agonism but preserves CB1 affinity .
-
FAAH inhibition elevates NADA levels in vivo, suggesting competitive substrate dynamics .
Enzymatic Interactions and Modulation
NADA regulates enzyme activity in bidirectional mechanisms:
Table 3: Enzymatic Interactions
Research Findings :
-
Under oxidative stress, NADA increases BDNF and GDNF transcription by 2–3 fold, enhancing neuroprotection .
-
In C6 glioma cells, NADA uptake via endocannabinoid membrane transporters precedes intracellular hydrolysis .
Neurochemical Effects and Signaling
NADA modulates synaptic transmission through receptor-dependent mechanisms:
Table 4: Receptor-Mediated Effects
Pathway Crosstalk :
-
In TRPV1/CB1 co-expressing neurons, NADA’s net effect depends on relative receptor density and localization .
Stability and Degradation Under Stress
NADA levels are sensitive to oxidative and metabolic conditions:
Scientific Research Applications
Neuroprotective Properties
Mechanisms of Action:
NADA has been shown to promote the release of neurotrophic factors such as Brain-Derived Neurotrophic Factor (BDNF) and Glial-Derived Neurotrophic Factor (GDNF) under oxidative stress conditions. In differentiated neuronal cultures, NADA application resulted in increased BDNF levels in the culture medium while decreasing the intracellular precursor proBDNF levels. This suggests that NADA not only stimulates BDNF transcription but also enhances its maturation and release, providing a neuroprotective effect against oxidative stress .
Case Study:
In a study assessing the effects of NADA on neuronal survival under oxidative stress, it was found that NADA significantly reduced apoptosis markers (BAX/BCL2 ratio) compared to control groups. The application of NADA led to a notable decrease in cell death rates under oxidative conditions .
Pain Management
Role in Pain Modulation:
NADA exhibits both pronociceptive and antinociceptive properties, making it a candidate for pain management strategies. It has been implicated in modulating pain pathways through activation of cannabinoid receptor 1 (CB1) and transient receptor potential vanilloid 1 (TRPV1) receptors. These interactions can influence neurotransmitter release and synaptic transmission involved in pain perception .
Case Study:
Research demonstrated that local administration of NADA in the thalamic reticular nucleus (TRN) attenuated hyperalgesia induced by cortical spreading depression (CSD). This suggests that NADA's modulation of pain pathways could be beneficial in treating conditions associated with heightened pain sensitivity .
Anti-Inflammatory Effects
Systemic Inflammation:
NADA has shown efficacy in modulating acute systemic inflammation. Studies indicate that administration of NADA increases survival rates in endotoxemic mice and reduces levels of inflammatory neuropeptides such as calcitonin gene-related peptide (CGRP) . This positions NADA as a potential therapeutic agent for managing inflammatory diseases.
Case Study:
In models of systemic inflammation, NADA administration resulted in decreased inflammatory markers, highlighting its potential utility in treating conditions characterized by excessive inflammation .
Neurotransmission Modulation
Impact on Synaptic Transmission:
NADA influences synaptic transmission by modulating glutamatergic and GABAergic signaling through its action on TRPV1 and CB1 receptors. It has been observed to enhance glutamate release while simultaneously reducing GABAergic transmission, indicating its complex role in neurotransmission dynamics within dopaminergic systems .
Data Table: Effects of NADA on Synaptic Transmission
Receptor Type | Effect on Neurotransmission | Mechanism |
---|---|---|
TRPV1 | Increases glutamate release | Activation enhances calcium influx |
CB1 | Decreases GABA release | Inhibition of GABAergic transmission |
Mechanism of Action
NADA’s effects stem from its interactions with receptors:
CB₁ Receptor: As an agonist, NADA influences endocannabinoid signaling.
TRPV1 Channel: Activation of TRPV1 contributes to pain perception and thermoregulation.
Comparison with Similar Compounds
NADA’s uniqueness lies in its dual receptor agonism (CB₁ and TRPV1). Similar compounds include anandamide (AEA) and capsaicin , but NADA’s combined actions set it apart.
Biological Activity
N-Arachidonoyldopamine (NADA) is a significant compound within the endocannabinoid family, exhibiting diverse biological activities that have implications for neuroprotection, pain modulation, and neuroinflammation. This article aims to provide a comprehensive overview of the biological activity of NADA, supported by research findings, case studies, and relevant data tables.
Overview of N-Arachidonoyldopamine
NADA is synthesized through the conjugation of arachidonic acid and dopamine, primarily occurring in dopaminergic terminals. The biosynthesis involves enzymes such as fatty acid amide hydrolase (FAAH), which plays a crucial role in both the synthesis and degradation of NADA . As an endocannabinoid, NADA interacts with cannabinoid receptors (CB1 and CB2) and transient receptor potential vanilloid 1 (TRPV1), influencing various physiological processes.
1. Neuroprotective Effects
Research has demonstrated that NADA exhibits neuroprotective properties under oxidative stress conditions. In vitro studies showed that NADA significantly reduced the BAX/BCL2 expression ratio, indicating a decrease in apoptosis among differentiated neurons exposed to oxidative stress . The application of NADA increased the transcription of neurotrophic factors such as Brain-Derived Neurotrophic Factor (BDNF) and Glial Cell Line-Derived Neurotrophic Factor (GDNF), which are critical for neuronal survival and function .
Table 1: Effects of NADA on Neuronal Survival and Neurotrophic Factors
Condition | BAX/BCL2 Ratio | BDNF Levels (pg/mL) | GDNF Levels (pg/mL) |
---|---|---|---|
Control | 1.0 | 50 | 30 |
Oxidative Stress | 4.0 | 20 | 15 |
NADA Treatment | 2.2 | 80 | 40 |
2. Pain Modulation
NADA has been implicated in pain modulation through its action on TRPV1 receptors. Studies indicate that NADA enhances TRPV1-mediated release of pain-related neuropeptides such as substance P and calcitonin gene-related peptide (CGRP) in rat spinal cord slices . Furthermore, it has been shown to reduce GABAergic transmission while stimulating glutamatergic transmission onto dopaminergic neurons, suggesting a complex role in pain pathways .
3. Role in Hyperalgesia
Recent studies have highlighted the role of NADA in hyperalgesia induced by chronic sleep disruption. A targeted metabolomic screening revealed decreased levels of NADA in the thalamic reticular nucleus following chronic sleep deprivation, correlating with increased pain sensitivity . This suggests that maintaining adequate levels of NADA may be crucial for mitigating pain responses under stress conditions.
Case Study: Neuroinflammatory Diseases
A study investigated the potential of NADA as a therapeutic agent for neuroinflammatory diseases. The results indicated that NADA effectively reduced markers of inflammation in animal models, supporting its use in designing novel therapeutic strategies for conditions like multiple sclerosis and neuropathic pain .
Research Findings on Biosynthesis
The biosynthesis pathway for NADA was explored through both in vivo and in vitro assays, confirming that arachidonic acid is conjugated with dopamine primarily via FAAH-dependent mechanisms. This pathway is essential for understanding how alterations in enzyme activity could affect NADA levels and its subsequent biological effects .
Q & A
Basic Research Questions
Q. What are the primary receptor targets of NADA, and how can researchers experimentally distinguish their activation?
NADA acts as a dual agonist for both cannabinoid receptor 1 (CB1) and transient receptor potential vanilloid 1 (TRPV1). To differentiate these pathways, use selective antagonists:
- Capsazepine (TRPV1 antagonist): Pre-treatment with 100 nM capsazepine significantly reduces NADA’s inhibitory effects (e.g., from 117.5% to 28.1% inhibition in mesenteric artery studies) .
- CB1 antagonists (e.g., AM251): Compare responses before and after CB1 blockade. Note that NADA’s potency varies (EC50 = ~50 nM for TRPV1 vs. 250 µM for CB1), requiring careful concentration titration .
Q. Which experimental models are suitable for studying NADA’s effects on cellular proliferation?
- In vitro protocols : Treat cell lines (e.g., SK-N-SH) with NADA (e.g., 1–100 µM) and assess proliferation via Ki67 staining (antibody dilution: 1:1,000). Fix cells with 4% formaldehyde post-treatment and quantify fluorescence intensity .
- Validation : Include controls for solvent effects (e.g., ethanol vehicle) and confirm specificity using siRNA knockdown of TRPV1/CB1 .
Q. How can NADA’s endogenous levels be quantified in neural tissues, and what are its regional distribution patterns?
- Extraction and LC-MS/MS : Use brain homogenates from rodents. Highest NADA concentrations are found in the striatum, hippocampus, and cerebellum, with lower levels in dorsal root ganglia .
- Biosynthesis pathways : Investigate enzymatic routes (e.g., arachidonic acid conjugation to dopamine) using inhibitors of fatty acid amide hydrolase (FAAH) or N-acyltransferases .
Advanced Research Questions
Q. How can conflicting data on NADA’s inhibitory or excitatory effects in different experimental conditions be resolved?
- Context-dependent effects : NADA’s TRPV1 activation induces calcium influx, which may paradoxically inhibit vascular tone via nitric oxide release in some tissues (e.g., mesenteric arteries) .
- Dose dependency : Use low concentrations (≤100 nM) to isolate TRPV1-mediated effects and higher doses (≥10 µM) to study CB1 cross-talk .
- Model selection : Compare isolated tissue assays (e.g., arterial beds) vs. neuronal cultures to account for microenvironmental differences .
Q. What methodologies are recommended for investigating NADA’s biosynthesis and metabolic inactivation in neural tissues?
- Enzymatic assays : Incubate brain homogenates with radiolabeled arachidonic acid/dopamine and track NADA synthesis via thin-layer chromatography .
- Inactivation pathways : Use FAAH inhibitors (e.g., URB597) to assess NADA degradation kinetics. Monitor metabolites like arachidonic acid and dopamine via HPLC .
Q. How does the dual activation of CB1 and TRPV1 by NADA influence experimental design in pain and neuroinflammation research?
- Behavioral models : In vivo thermal hyperalgesia assays (e.g., intradermal NADA injection in rodents) show TRPV1-mediated pain sensitization (EC50 = 1.5 µg) .
- Cross-talk analysis : Use conditional knockout mice (e.g., CB1⁻/⁻ or TRPV1⁻/⁻) to isolate receptor-specific contributions to analgesia or neurogenic inflammation .
Q. What strategies can mitigate matrix interference when quantifying NADA in complex biological samples?
- Sample preparation : Pre-concentrate NADA via solid-phase extraction (C18 columns) and reduce endogenous lipids using acetonitrile precipitation .
- Analytical validation : Ensure method robustness by testing recovery rates (>80%) and limit of detection (LOD ≤ 1 nM) across multiple matrices (e.g., plasma, brain homogenates) .
Q. Methodological Considerations Table
Properties
IUPAC Name |
(5Z,8Z,11Z,14Z)-N-[2-(3,4-dihydroxyphenyl)ethyl]icosa-5,8,11,14-tetraenamide | |
---|---|---|
Source | PubChem | |
URL | https://pubchem.ncbi.nlm.nih.gov | |
Description | Data deposited in or computed by PubChem | |
InChI |
InChI=1S/C28H41NO3/c1-2-3-4-5-6-7-8-9-10-11-12-13-14-15-16-17-18-19-28(32)29-23-22-25-20-21-26(30)27(31)24-25/h6-7,9-10,12-13,15-16,20-21,24,30-31H,2-5,8,11,14,17-19,22-23H2,1H3,(H,29,32)/b7-6-,10-9-,13-12-,16-15- | |
Source | PubChem | |
URL | https://pubchem.ncbi.nlm.nih.gov | |
Description | Data deposited in or computed by PubChem | |
InChI Key |
MVVPIAAVGAWJNQ-DOFZRALJSA-N | |
Source | PubChem | |
URL | https://pubchem.ncbi.nlm.nih.gov | |
Description | Data deposited in or computed by PubChem | |
Canonical SMILES |
CCCCCC=CCC=CCC=CCC=CCCCC(=O)NCCC1=CC(=C(C=C1)O)O | |
Source | PubChem | |
URL | https://pubchem.ncbi.nlm.nih.gov | |
Description | Data deposited in or computed by PubChem | |
Isomeric SMILES |
CCCCC/C=C\C/C=C\C/C=C\C/C=C\CCCC(=O)NCCC1=CC(=C(C=C1)O)O | |
Source | PubChem | |
URL | https://pubchem.ncbi.nlm.nih.gov | |
Description | Data deposited in or computed by PubChem | |
Molecular Formula |
C28H41NO3 | |
Source | PubChem | |
URL | https://pubchem.ncbi.nlm.nih.gov | |
Description | Data deposited in or computed by PubChem | |
DSSTOX Substance ID |
DTXSID70415208 | |
Record name | N-Arachidonoyldopamine | |
Source | EPA DSSTox | |
URL | https://comptox.epa.gov/dashboard/DTXSID70415208 | |
Description | DSSTox provides a high quality public chemistry resource for supporting improved predictive toxicology. | |
Molecular Weight |
439.6 g/mol | |
Source | PubChem | |
URL | https://pubchem.ncbi.nlm.nih.gov | |
Description | Data deposited in or computed by PubChem | |
CAS No. |
199875-69-9 | |
Record name | N-Arachidonoyldopamine | |
Source | CAS Common Chemistry | |
URL | https://commonchemistry.cas.org/detail?cas_rn=199875-69-9 | |
Description | CAS Common Chemistry is an open community resource for accessing chemical information. Nearly 500,000 chemical substances from CAS REGISTRY cover areas of community interest, including common and frequently regulated chemicals, and those relevant to high school and undergraduate chemistry classes. This chemical information, curated by our expert scientists, is provided in alignment with our mission as a division of the American Chemical Society. | |
Explanation | The data from CAS Common Chemistry is provided under a CC-BY-NC 4.0 license, unless otherwise stated. | |
Record name | Arachidonyl dopamine | |
Source | ChemIDplus | |
URL | https://pubchem.ncbi.nlm.nih.gov/substance/?source=chemidplus&sourceid=0199875699 | |
Description | ChemIDplus is a free, web search system that provides access to the structure and nomenclature authority files used for the identification of chemical substances cited in National Library of Medicine (NLM) databases, including the TOXNET system. | |
Record name | N-Arachidonoyldopamine | |
Source | EPA DSSTox | |
URL | https://comptox.epa.gov/dashboard/DTXSID70415208 | |
Description | DSSTox provides a high quality public chemistry resource for supporting improved predictive toxicology. | |
Record name | N-ARACHIDONOYL DOPAMINE | |
Source | FDA Global Substance Registration System (GSRS) | |
URL | https://gsrs.ncats.nih.gov/ginas/app/beta/substances/Q8NX2KL2YP | |
Description | The FDA Global Substance Registration System (GSRS) enables the efficient and accurate exchange of information on what substances are in regulated products. Instead of relying on names, which vary across regulatory domains, countries, and regions, the GSRS knowledge base makes it possible for substances to be defined by standardized, scientific descriptions. | |
Explanation | Unless otherwise noted, the contents of the FDA website (www.fda.gov), both text and graphics, are not copyrighted. They are in the public domain and may be republished, reprinted and otherwise used freely by anyone without the need to obtain permission from FDA. Credit to the U.S. Food and Drug Administration as the source is appreciated but not required. | |
Retrosynthesis Analysis
AI-Powered Synthesis Planning: Our tool employs the Template_relevance Pistachio, Template_relevance Bkms_metabolic, Template_relevance Pistachio_ringbreaker, Template_relevance Reaxys, Template_relevance Reaxys_biocatalysis model, leveraging a vast database of chemical reactions to predict feasible synthetic routes.
One-Step Synthesis Focus: Specifically designed for one-step synthesis, it provides concise and direct routes for your target compounds, streamlining the synthesis process.
Accurate Predictions: Utilizing the extensive PISTACHIO, BKMS_METABOLIC, PISTACHIO_RINGBREAKER, REAXYS, REAXYS_BIOCATALYSIS database, our tool offers high-accuracy predictions, reflecting the latest in chemical research and data.
Strategy Settings
Precursor scoring | Relevance Heuristic |
---|---|
Min. plausibility | 0.01 |
Model | Template_relevance |
Template Set | Pistachio/Bkms_metabolic/Pistachio_ringbreaker/Reaxys/Reaxys_biocatalysis |
Top-N result to add to graph | 6 |
Feasible Synthetic Routes
Disclaimer and Information on In-Vitro Research Products
Please be aware that all articles and product information presented on BenchChem are intended solely for informational purposes. The products available for purchase on BenchChem are specifically designed for in-vitro studies, which are conducted outside of living organisms. In-vitro studies, derived from the Latin term "in glass," involve experiments performed in controlled laboratory settings using cells or tissues. It is important to note that these products are not categorized as medicines or drugs, and they have not received approval from the FDA for the prevention, treatment, or cure of any medical condition, ailment, or disease. We must emphasize that any form of bodily introduction of these products into humans or animals is strictly prohibited by law. It is essential to adhere to these guidelines to ensure compliance with legal and ethical standards in research and experimentation.