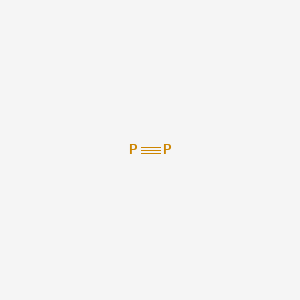
Diphosphorus
- Click on QUICK INQUIRY to receive a quote from our team of experts.
- With the quality product at a COMPETITIVE price, you can focus more on your research.
Description
Diphosphorus is an inorganic chemical with the chemical formula P2 . Unlike nitrogen, its lighter pnictogen neighbor which forms a stable N2 molecule with a nitrogen to nitrogen triple bond, phosphorus prefers a tetrahedral form P4 because P-P pi-bonds are high in energy . Diphosphorus is, therefore, very reactive with a bond-dissociation energy (117 kcal/mol or 490 kJ/mol) half that of dinitrogen .
Synthesis Analysis
Diphosphorus has been generated by heating white phosphorus at 1100 kelvins (827 °C) . Some advancements have been obtained in generating the diatomic molecule in homogeneous solution under normal conditions with the use of some transition metal complexes . Methods for dissociation of bonds in P4 molecules via photoexcitation were also proposed .Molecular Structure Analysis
The bond distance in diphosphorus has been measured at 1.8934 Å . It is thermodynamically stable above 1200 °C and until 2000 °C .Chemical Reactions Analysis
Diphosphorus is highly reactive and can form various compounds. For instance, it reacts with oxygen to form diphosphorus pentoxide .Physical And Chemical Properties Analysis
Diphosphorus is a gaseous form of phosphorus that is thermodynamically stable above 1200 °C and until 2000 °C . It is very reactive with a bond-dissociation energy (117 kcal/mol or 490 kJ/mol) half that of dinitrogen .Scientific Research Applications
Asymmetric Hydrogenation and Chirality
Diphosphorus has significant applications in asymmetric hydrogenation, which is crucial in producing chiral compounds. C1-symmetric diphosphorus ligands have shown remarkable effectiveness in this area, often outperforming their C2-symmetric counterparts. This method is valuable for creating pharmaceutical intermediates, bioactive molecules, drugs, agrochemicals, and fragrances. However, the reliance on precious metals in current methods poses challenges, emphasizing the need for alternatives involving base or earth-abundant metals (Sen & Chikkali, 2021).
Reactivity and Isolation
Diphosphorus is highly reactive and traditionally studied only in gas phases or inert gas matrices. Recent work has led to the isolation of metal complexes of neutral diphosphorus, analogous to metal dinitrogen compounds. This opens new avenues for exploring diphosphorus' properties and applications (Abbenseh & Goicoechea, 2021).
Generation and Capture in Homogeneous Solution
Diphosphorus can be generated in a mild manner from a niobium complex and trapped efficiently by organic dienes to produce organodiphosphorus compounds. This process allows for easier handling and application of diphosphorus in a range of reactions (Piro, Figueroa, McKellar, & Cummins, 2006).
Catalytic Applications
Small bite-angle diphosphorus ligands with single-atom linkers have found growing use in catalysis. These ligands are employed in various catalytic transformations of alkenes, including ethene oligomerization, polymerization, hydroacylation, hydrogenation, transfer hydrogenation, and hydrogen-borrowing reactions (Mansell, 2017).
Stabilization and Bonding
Studies on stabilizing diphosphorus reveal unique properties, such as its ability to act as a bridging ligand in its neutral, triply bonded form. This stabilization, achieved by coordination to metal fragments, is significant for controlled release and utilization of diphosphorus in various applications (Sun et al., 2021).
Electronic Devices
Organophosphorus derivatives, including diphosphorus compounds, are essential in manufacturing electronic devices. Their applications span across OLEDs, photovoltaic cells, DSSCs, OFETs, and electrochromic cells, demonstrating the versatility of diphosphorus in advanced technological applications (Joly, Bouit, & Hissler, 2016).
properties
IUPAC Name |
phosphanylidynephosphane |
Source
|
---|---|---|
Source | PubChem | |
URL | https://pubchem.ncbi.nlm.nih.gov | |
Description | Data deposited in or computed by PubChem | |
InChI |
InChI=1S/P2/c1-2 |
Source
|
Source | PubChem | |
URL | https://pubchem.ncbi.nlm.nih.gov | |
Description | Data deposited in or computed by PubChem | |
InChI Key |
FOBPTJZYDGNHLR-UHFFFAOYSA-N |
Source
|
Source | PubChem | |
URL | https://pubchem.ncbi.nlm.nih.gov | |
Description | Data deposited in or computed by PubChem | |
Canonical SMILES |
P#P |
Source
|
Source | PubChem | |
URL | https://pubchem.ncbi.nlm.nih.gov | |
Description | Data deposited in or computed by PubChem | |
Molecular Formula |
P2 |
Source
|
Source | PubChem | |
URL | https://pubchem.ncbi.nlm.nih.gov | |
Description | Data deposited in or computed by PubChem | |
DSSTOX Substance ID |
DTXSID301027109 |
Source
|
Record name | Phosphanylidynephosphane | |
Source | EPA DSSTox | |
URL | https://comptox.epa.gov/dashboard/DTXSID301027109 | |
Description | DSSTox provides a high quality public chemistry resource for supporting improved predictive toxicology. | |
Molecular Weight |
61.94752400 g/mol |
Source
|
Source | PubChem | |
URL | https://pubchem.ncbi.nlm.nih.gov | |
Description | Data deposited in or computed by PubChem | |
Product Name |
Diphosphorus | |
CAS RN |
12185-09-0 |
Source
|
Record name | Diphosphorus | |
Source | CAS Common Chemistry | |
URL | https://commonchemistry.cas.org/detail?cas_rn=12185-09-0 | |
Description | CAS Common Chemistry is an open community resource for accessing chemical information. Nearly 500,000 chemical substances from CAS REGISTRY cover areas of community interest, including common and frequently regulated chemicals, and those relevant to high school and undergraduate chemistry classes. This chemical information, curated by our expert scientists, is provided in alignment with our mission as a division of the American Chemical Society. | |
Explanation | The data from CAS Common Chemistry is provided under a CC-BY-NC 4.0 license, unless otherwise stated. | |
Record name | Phosphanylidynephosphane | |
Source | EPA DSSTox | |
URL | https://comptox.epa.gov/dashboard/DTXSID301027109 | |
Description | DSSTox provides a high quality public chemistry resource for supporting improved predictive toxicology. | |
Disclaimer and Information on In-Vitro Research Products
Please be aware that all articles and product information presented on BenchChem are intended solely for informational purposes. The products available for purchase on BenchChem are specifically designed for in-vitro studies, which are conducted outside of living organisms. In-vitro studies, derived from the Latin term "in glass," involve experiments performed in controlled laboratory settings using cells or tissues. It is important to note that these products are not categorized as medicines or drugs, and they have not received approval from the FDA for the prevention, treatment, or cure of any medical condition, ailment, or disease. We must emphasize that any form of bodily introduction of these products into humans or animals is strictly prohibited by law. It is essential to adhere to these guidelines to ensure compliance with legal and ethical standards in research and experimentation.