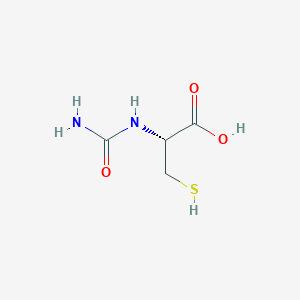
N-Carbamoyl-L-cysteine
Overview
Description
N-Carbamoyl-L-cysteine is a derivative of the amino acid L-cysteine, characterized by the presence of a carbamoyl group attached to the nitrogen atom of the cysteine molecule.
Preparation Methods
Synthetic Routes and Reaction Conditions: N-Carbamoyl-L-cysteine can be synthesized through the bioconversion of D,L-2-amino-Δ2-thiazoline-4-carboxylic acid (D,L-ATC) using specific bacterial strains such as Pseudomonas sp. The process involves three main steps:
Racemization: Conversion of D-ATC to L-ATC by ATC racemase.
Ring-Opening Reaction: L-ATC is converted to this compound by L-ATC hydrolase.
Hydrolysis: this compound is hydrolyzed to L-cysteine by this compound amidohydrolase.
Industrial Production Methods: Industrial production of this compound often involves microbial fermentation processes. Bacteria such as Pseudomonas sp. are genetically engineered to enhance the production yield. The fermentation process is optimized by controlling factors such as pH, temperature, and nutrient availability .
Chemical Reactions Analysis
Types of Reactions: N-Carbamoyl-L-cysteine undergoes various chemical reactions, including:
Hydrolysis: Conversion to L-cysteine.
Oxidation: Formation of disulfide bonds.
Substitution: Reaction with other amino acids or peptides.
Common Reagents and Conditions:
Hydrolysis: Catalyzed by specific enzymes such as this compound amidohydrolase.
Oxidation: Typically involves oxidizing agents like hydrogen peroxide.
Substitution: Requires specific catalysts and reaction conditions depending on the desired product.
Major Products:
L-Cysteine: A key product formed through hydrolysis.
Disulfide Compounds: Formed through oxidation reactions.
Scientific Research Applications
Biosynthesis of L-Cysteine
N-Carbamoyl-L-cysteine serves as an important precursor in the enzymatic conversion processes leading to L-cysteine production. The synthesis of L-cysteine from various substrates often involves NCC as an intermediate, particularly in microbial fermentation processes.
Enzymatic Conversion Pathways
The enzymatic pathway for converting DL-2-amino-Δ2-thiazoline-4-carboxylic acid (DL-ATC) to L-cysteine includes several key steps where NCC is utilized:
- Racemization : Conversion of D-ATC to L-ATC by ATC racemase.
- Hydrolysis : Ring-opening of L-ATC by L-ATC hydrolase to produce this compound.
- Final Hydrolysis : Hydrolysis of this compound to yield L-cysteine via S-carbamoyl-L-cysteine hydrolase .
This process has been optimized in various studies, achieving significant yields of L-cysteine through metabolic engineering techniques.
Pharmaceutical Industry
This compound is utilized in the pharmaceutical industry as a precursor for synthesizing drugs and nutraceuticals. Its role as a sulfur source is critical in developing compounds that require thiol groups for biological activity.
Food Industry
In food technology, NCC is explored for its potential as a food additive and flavor enhancer due to its ability to influence the sulfur content in food products, enhancing taste profiles and nutritional value .
Cosmetic Industry
The cosmetic sector also benefits from this compound, particularly in formulations aimed at hair treatment. It acts as a substitute for thioglycolic acid in permanent waving solutions, breaking disulfide bonds in keratin .
Case Studies and Research Findings
Recent advances in metabolic engineering have led to the development of recombinant strains of E. coli capable of producing higher yields of L-cysteine through the strategic expression of genes involved in sulfur metabolism and NCC utilization. For instance, engineered strains have achieved production levels exceeding 11 g/L of L-cysteine .
Challenges and Future Directions
While the applications of this compound are promising, challenges remain in optimizing production processes and ensuring economic viability. Future research may focus on:
- Enhancing enzyme stability and activity under industrial conditions.
- Exploring alternative microbial strains for better NCC utilization.
- Investigating the environmental impacts of large-scale NCC production.
Mechanism of Action
The mechanism of action of N-carbamoyl-L-cysteine involves its conversion to L-cysteine, which plays a crucial role in various biochemical processes. L-cysteine is a precursor for the synthesis of glutathione, an important antioxidant. The conversion process involves enzymes such as ATC racemase, L-ATC hydrolase, and this compound amidohydrolase .
Comparison with Similar Compounds
N-Acetyl-L-cysteine: Another derivative of L-cysteine with a different functional group.
L-Cystine: The oxidized dimer form of L-cysteine.
S-Carbamoyl-L-cysteine: A similar compound with a different carbamoyl group attachment.
Uniqueness: Its role as an intermediate in the bioconversion process to L-cysteine sets it apart from other cysteine derivatives .
Biological Activity
N-Carbamoyl-L-cysteine (NCC) is a derivative of L-cysteine that has garnered attention for its potential biological activities, particularly in enzyme inhibition and as a precursor in metabolic pathways. This article delves into the biological properties of NCC, highlighting its mechanisms of action, therapeutic potential, and relevant research findings.
1. Mechanisms of Biological Activity
Enzyme Inhibition
this compound has been identified as an inhibitor of several key enzymes, notably urease and cysteine proteases. Urease plays a significant role in the metabolism of urea, and its inhibition is crucial in conditions like hyperammonemia. Studies indicate that NCC effectively inhibits urease activity, suggesting its potential therapeutic role in managing elevated ammonia levels in the blood.
Cysteine proteases, such as papain and cathepsin B, are involved in various physiological processes and disease states. The inhibition of these enzymes by NCC may provide avenues for therapeutic applications in conditions characterized by their dysregulation.
Biosynthesis of L-Cysteine
NCC serves as a precursor in the biosynthesis of L-cysteine, an amino acid essential for protein synthesis, antioxidant defense, and detoxification processes. The conversion of NCC to L-cysteine is facilitated by specific enzymes, such as S-carbamoyl-L-cysteine hydrolase, which operate in microbial systems. This transformation is vital for maintaining cellular redox status and modulating signaling pathways due to the role of L-cysteine in glutathione synthesis.
2.1 Antitumoral Activity
A study investigated the antitumoral effects of S-carbamoyl-L-cysteine derivatives, including NCC. The research demonstrated that ethyl and chloroethyl derivatives exhibited significant curative effects on specific animal tumors. Interestingly, modifications to the NCC structure enhanced its chemotherapeutic effectiveness without increasing toxicity, indicating a unique mechanism distinct from traditional cytostatics .
2.2 Enzymatic Characterization
Research on the enzymatic properties of NCC revealed insights into its specificity and activity profiles. For instance, studies have shown that certain bacterial enzymes exhibit high activity toward NCC when utilized in biotransformation processes. This highlights the potential for industrial applications where NCC can be leveraged for producing valuable amino acids through enzymatic pathways .
3. Comparative Data Table
The following table summarizes key findings related to the biological activity of this compound:
4. Conclusion
This compound exhibits significant biological activity through its roles in enzyme inhibition and as a precursor to L-cysteine. Its potential therapeutic applications span from managing hyperammonemia to contributing to cancer treatment strategies. Ongoing research continues to uncover the diverse biochemical interactions and metabolic pathways associated with NCC, paving the way for innovative applications in medicine and biotechnology.
Q & A
Basic Research Questions
Q. What are the standard synthetic routes for preparing N-Carbamoyl-L-cysteine, and how are intermediates characterized?
NCC is synthesized via carbamoylation of L-cysteine or its derivatives. A common method involves reacting N-acetyl-L-cysteine (NAC) with substituted phenylisocyanates under controlled conditions (e.g., acetone as solvent, room temperature). Key intermediates are characterized using H NMR, C NMR, IR spectroscopy, and mass spectrometry to confirm structural integrity and purity. For example, H NMR in acetone-d typically shows signals for the acetyl group ( ppm) and carbamoyl protons ( ppm) .
Q. What analytical techniques are recommended for assessing NCC purity and stability in aqueous solutions?
High-performance liquid chromatography (HPLC) with UV detection ( nm) is widely used for purity assessment. Lyophilized NCC should be stored at C to prevent hydrolysis. Stability studies should include pH-dependent degradation assays, monitored via spectrophotometry or LC-MS to identify breakdown products like L-cysteine or carbamic acid derivatives .
Q. How is NCC’s role in microbial cysteine biosynthesis experimentally validated?
NCC is an intermediate in the conversion of D,L-2-amino--thiazoline-4-carboxylic acid (DL-ATC) to L-cysteine in Pseudomonas spp. Knockout strains lacking NCC amidohydrolase activity (e.g., Pseudomonas sp. ON-4a) are used to confirm its metabolic role via enzyme assays and isotopic labeling. Enzymatic activity is quantified by measuring L-cysteine production using Ellman’s reagent (DTNB) .
Advanced Research Questions
Q. How can contradictory data on NCC amidohydrolase substrate specificity be resolved?
Discrepancies in substrate affinity (e.g., for NCC vs. other N-carbamoyl-amino acids) may arise from enzyme isoforms or assay conditions. To resolve these:
- Perform kinetic studies (, ) under standardized pH and temperature.
- Use X-ray crystallography or cryo-EM to compare active-site geometries of homologs (e.g., Rhizobium radiobacter vs. Pseudomonas sp. enzymes).
- Validate findings with site-directed mutagenesis targeting residues implicated in substrate binding (e.g., Arg-134 in Pseudomonas NCC amidohydrolase) .
Q. What strategies optimize NCC derivatization for enhanced biological activity while minimizing side reactions?
To improve selectivity in NCC derivatization (e.g., for antioxidant or chelating applications):
- Use electron-deficient isocyanates (e.g., o-nitro-p-trifluoromethylphenylisocyanate) to reduce competing hydrolysis.
- Introduce protecting groups (e.g., tert-butyloxycarbonyl, Boc) on the cysteine thiol prior to carbamoylation.
- Monitor reaction progress in real-time via inline FTIR to detect isocyanate consumption ( cm) .
Q. How should researchers design experiments to distinguish NCC’s direct antioxidant effects from its role as a cysteine prodrug?
- Treat cell cultures with NCC and measure intracellular glutathione (GSH) levels via HPLC or fluorescent probes (e.g., monochlorobimane).
- Use a cysteine-free medium and compare results with exogenous L-cysteine supplementation.
- Inhibit enzymatic NCC hydrolysis (e.g., with EDTA to chelate metal-dependent amidohydrolases) and assess residual antioxidant capacity via DPPH or ABTS assays .
Q. What computational approaches predict NCC’s interaction with biological targets, such as redox-sensitive transcription factors?
- Perform molecular docking (e.g., AutoDock Vina) using NCC’s 3D structure (PubChem CID: 24583) and target proteins (e.g., Nrf2-Keap1 complex).
- Validate predictions with surface plasmon resonance (SPR) to measure binding kinetics (, ).
- Use QM/MM simulations to model thiol-disulfide exchange mechanisms in NCC’s antioxidant activity .
Q. Methodological Best Practices
Q. How should researchers address variability in NCC amidohydrolase activity across microbial strains?
- Include positive controls (e.g., E. coli expressing recombinant Pseudomonas NCC amidohydrolase) and negative controls (vector-only strains).
- Normalize activity to total protein concentration (Bradford assay) and report specific activity (µmol·min·mg).
- Replicate experiments across independent cultures () and use ANOVA with post-hoc Tukey tests for statistical rigor .
Q. What experimental designs mitigate confounding factors in NCC’s in vivo pharmacokinetic studies?
- Use radiolabeled C-NCC to track absorption/distribution without interference from endogenous cysteine.
- Collect timed blood/tissue samples and analyze via scintillation counting coupled with HPLC to distinguish NCC from metabolites.
- Control for diet-induced cysteine fluctuations by using defined rodent chow .
Properties
IUPAC Name |
(2R)-2-(carbamoylamino)-3-sulfanylpropanoic acid | |
---|---|---|
Source | PubChem | |
URL | https://pubchem.ncbi.nlm.nih.gov | |
Description | Data deposited in or computed by PubChem | |
InChI |
InChI=1S/C4H8N2O3S/c5-4(9)6-2(1-10)3(7)8/h2,10H,1H2,(H,7,8)(H3,5,6,9)/t2-/m0/s1 | |
Source | PubChem | |
URL | https://pubchem.ncbi.nlm.nih.gov | |
Description | Data deposited in or computed by PubChem | |
InChI Key |
APFSAMXTZRYBKF-REOHCLBHSA-N | |
Source | PubChem | |
URL | https://pubchem.ncbi.nlm.nih.gov | |
Description | Data deposited in or computed by PubChem | |
Canonical SMILES |
C(C(C(=O)O)NC(=O)N)S | |
Source | PubChem | |
URL | https://pubchem.ncbi.nlm.nih.gov | |
Description | Data deposited in or computed by PubChem | |
Isomeric SMILES |
C([C@@H](C(=O)O)NC(=O)N)S | |
Source | PubChem | |
URL | https://pubchem.ncbi.nlm.nih.gov | |
Description | Data deposited in or computed by PubChem | |
Molecular Formula |
C4H8N2O3S | |
Source | PubChem | |
URL | https://pubchem.ncbi.nlm.nih.gov | |
Description | Data deposited in or computed by PubChem | |
DSSTOX Substance ID |
DTXSID90947455 | |
Record name | N-[Hydroxy(imino)methyl]cysteine | |
Source | EPA DSSTox | |
URL | https://comptox.epa.gov/dashboard/DTXSID90947455 | |
Description | DSSTox provides a high quality public chemistry resource for supporting improved predictive toxicology. | |
Molecular Weight |
164.19 g/mol | |
Source | PubChem | |
URL | https://pubchem.ncbi.nlm.nih.gov | |
Description | Data deposited in or computed by PubChem | |
CAS No. |
24583-23-1 | |
Record name | N-(Aminocarbonyl)-L-cysteine | |
Source | CAS Common Chemistry | |
URL | https://commonchemistry.cas.org/detail?cas_rn=24583-23-1 | |
Description | CAS Common Chemistry is an open community resource for accessing chemical information. Nearly 500,000 chemical substances from CAS REGISTRY cover areas of community interest, including common and frequently regulated chemicals, and those relevant to high school and undergraduate chemistry classes. This chemical information, curated by our expert scientists, is provided in alignment with our mission as a division of the American Chemical Society. | |
Explanation | The data from CAS Common Chemistry is provided under a CC-BY-NC 4.0 license, unless otherwise stated. | |
Record name | N-Carbamoyl-L-cysteine | |
Source | ChemIDplus | |
URL | https://pubchem.ncbi.nlm.nih.gov/substance/?source=chemidplus&sourceid=0024583231 | |
Description | ChemIDplus is a free, web search system that provides access to the structure and nomenclature authority files used for the identification of chemical substances cited in National Library of Medicine (NLM) databases, including the TOXNET system. | |
Record name | N-[Hydroxy(imino)methyl]cysteine | |
Source | EPA DSSTox | |
URL | https://comptox.epa.gov/dashboard/DTXSID90947455 | |
Description | DSSTox provides a high quality public chemistry resource for supporting improved predictive toxicology. | |
Record name | N-carbamoyl-L-cysteine | |
Source | European Chemicals Agency (ECHA) | |
URL | https://echa.europa.eu/substance-information/-/substanceinfo/100.042.102 | |
Description | The European Chemicals Agency (ECHA) is an agency of the European Union which is the driving force among regulatory authorities in implementing the EU's groundbreaking chemicals legislation for the benefit of human health and the environment as well as for innovation and competitiveness. | |
Explanation | Use of the information, documents and data from the ECHA website is subject to the terms and conditions of this Legal Notice, and subject to other binding limitations provided for under applicable law, the information, documents and data made available on the ECHA website may be reproduced, distributed and/or used, totally or in part, for non-commercial purposes provided that ECHA is acknowledged as the source: "Source: European Chemicals Agency, http://echa.europa.eu/". Such acknowledgement must be included in each copy of the material. ECHA permits and encourages organisations and individuals to create links to the ECHA website under the following cumulative conditions: Links can only be made to webpages that provide a link to the Legal Notice page. | |
Retrosynthesis Analysis
AI-Powered Synthesis Planning: Our tool employs the Template_relevance Pistachio, Template_relevance Bkms_metabolic, Template_relevance Pistachio_ringbreaker, Template_relevance Reaxys, Template_relevance Reaxys_biocatalysis model, leveraging a vast database of chemical reactions to predict feasible synthetic routes.
One-Step Synthesis Focus: Specifically designed for one-step synthesis, it provides concise and direct routes for your target compounds, streamlining the synthesis process.
Accurate Predictions: Utilizing the extensive PISTACHIO, BKMS_METABOLIC, PISTACHIO_RINGBREAKER, REAXYS, REAXYS_BIOCATALYSIS database, our tool offers high-accuracy predictions, reflecting the latest in chemical research and data.
Strategy Settings
Precursor scoring | Relevance Heuristic |
---|---|
Min. plausibility | 0.01 |
Model | Template_relevance |
Template Set | Pistachio/Bkms_metabolic/Pistachio_ringbreaker/Reaxys/Reaxys_biocatalysis |
Top-N result to add to graph | 6 |
Feasible Synthetic Routes
Disclaimer and Information on In-Vitro Research Products
Please be aware that all articles and product information presented on BenchChem are intended solely for informational purposes. The products available for purchase on BenchChem are specifically designed for in-vitro studies, which are conducted outside of living organisms. In-vitro studies, derived from the Latin term "in glass," involve experiments performed in controlled laboratory settings using cells or tissues. It is important to note that these products are not categorized as medicines or drugs, and they have not received approval from the FDA for the prevention, treatment, or cure of any medical condition, ailment, or disease. We must emphasize that any form of bodily introduction of these products into humans or animals is strictly prohibited by law. It is essential to adhere to these guidelines to ensure compliance with legal and ethical standards in research and experimentation.