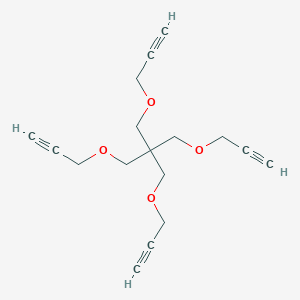
Tetrakis(2-propynyloxymethyl) methane
Overview
Description
Tetrakis(2-propynyloxymethyl) methane is a chemical compound with the molecular formula C17H20O4 . It is used in the preparation of Aminoglycoside Cyclic Oligosaccharides as Multimeric P- and E-selectin antagonists .
Synthesis Analysis
A series of amphiphilic conetworks (APCNs) with well-defined molecular structures were prepared via a copper-catalyzed 1,3-dipolar azide–alkyne cycloaddition (CuAAC) of tetrakis (2-propynyloxymethyl)methane (TMOP), diazide end-functionalized triblock copolymers of poly (ε-caprolactone) with poly (ethylene glycol) (N3-PCL-PEG-PCL-N3) .Molecular Structure Analysis
The molecular formula of this compound is C17H20O4 . The IUPAC name of this compound is 1,3-bis(prop-2-ynoxy)-2,2-bis(prop-2-ynoxymethyl)propane . The InChI of this compound is InChI=1S/C17H20O4/c1-5-9-18-13-17(14-19-10-6-2,15-20-11-7-3)16-21-12-8-4/h1-4H,9-16H2 .Chemical Reactions Analysis
Tetrakis(2-propynyloxymethyl)methane is used in the synthesis of Aminoglycoside Cyclic Oligosaccharides .Physical and Chemical Properties Analysis
The molecular weight of Tetrakis(2-propynyloxymethyl)methane is 288.34 g/mol . It has a melting point of >47°C (dec.) . It is slightly soluble in Chloroform and Ethyl Acetate when sonicated . It is a solid substance that ranges in color from Light Orange to Very Dark Brown Waxy .Scientific Research Applications
Synthesis and Structural Studies
- Synthesis Techniques : Tetrakis(2-propynyloxymethyl) methane has been synthesized through nucleophilic aromatic substitution and other methods, contributing to the development of new chemical compounds and families (Matsumoto, Kannami, & Oda, 2003).
- Crystal Structure Analysis : Studies involving this compound and related compounds often focus on elucidating their molecular structures, which is crucial for understanding their physical and chemical properties (Matsumoto et al., 2002).
Material Science and Engineering
- Construction of Metal-Organic Frameworks : This compound has been used to create highly porous metal-organic frameworks (MOFs), which are valuable for gas adsorption and separation, indicating potential applications in environmental and material sciences (Lin et al., 2013).
- Photoluminescent Materials : Its derivatives have been synthesized for use in light-emitting devices, underscoring the importance of these compounds in developing advanced photoluminescent materials (Yeh et al., 2001).
Chemical Reactivity and Applications
- Chemical Reactions and Transformations : Research has explored the reactivity of this compound in various chemical reactions, leading to the synthesis of new compounds with unique properties, such as benzannulation products (Quast, Nieger, & Dötz, 2000).
- Formation of Inclusion Compounds : Studies have examined the ability of these compounds to form inclusion compounds with different solvents, providing insights into their potential use in host-guest chemistry applications (Thaimattam et al., 2001).
Electronics and Optoelectronics
- Use in Electronic Devices : Derivatives of this compound have been designed for hole-transport applications in organic light-emitting diodes (OLEDs), highlighting their significance in the field of electronics (Zhao, Tanjutco, & Thayumanavan, 2001).
Mechanism of Action
Target of Action
Tetrakis(2-propynyloxymethyl) methane is primarily used in the preparation of Aminoglycoside Cyclic Oligosaccharides . These oligosaccharides serve as multimeric P- and E-selectin antagonists , which play a crucial role in the adhesion of leukocytes to endothelial cells, a key step in inflammation.
Mode of Action
The compound interacts with its targets through a copper-catalyzed 1,3-dipolar azide–alkyne cycloaddition (CuAAC) . This reaction involves the coupling of an azide and an alkyne to form a triazole ring, a process that is often used in click chemistry .
Biochemical Pathways
The biochemical pathways affected by this compound are primarily related to the formation of hydrogels . The compound is used in the synthesis of PEG-based single-chain hydrogels , which are known for their high mechanical strength and biodegradability .
Pharmacokinetics
It’s known that the compound isslightly soluble in chloroform and ethyl acetate
Result of Action
The primary result of the action of this compound is the formation of high-strength, biodegradable hydrogels . These hydrogels have potential applications in artificial medical devices or scaffolding materials for regenerative medicine .
Action Environment
The action of this compound can be influenced by environmental factors such as temperature and solvent. For instance, the compound has a melting point of >47°C , suggesting that it is stable at room temperature but may degrade at higher temperatures. Additionally, the compound’s solubility in different solvents can affect its reactivity and efficacy .
Properties
IUPAC Name |
1,3-bis(prop-2-ynoxy)-2,2-bis(prop-2-ynoxymethyl)propane | |
---|---|---|
Source | PubChem | |
URL | https://pubchem.ncbi.nlm.nih.gov | |
Description | Data deposited in or computed by PubChem | |
InChI |
InChI=1S/C17H20O4/c1-5-9-18-13-17(14-19-10-6-2,15-20-11-7-3)16-21-12-8-4/h1-4H,9-16H2 | |
Source | PubChem | |
URL | https://pubchem.ncbi.nlm.nih.gov | |
Description | Data deposited in or computed by PubChem | |
InChI Key |
PBIYZQCDULGYAW-UHFFFAOYSA-N | |
Source | PubChem | |
URL | https://pubchem.ncbi.nlm.nih.gov | |
Description | Data deposited in or computed by PubChem | |
Canonical SMILES |
C#CCOCC(COCC#C)(COCC#C)COCC#C | |
Source | PubChem | |
URL | https://pubchem.ncbi.nlm.nih.gov | |
Description | Data deposited in or computed by PubChem | |
Molecular Formula |
C17H20O4 | |
Source | PubChem | |
URL | https://pubchem.ncbi.nlm.nih.gov | |
Description | Data deposited in or computed by PubChem | |
Molecular Weight |
288.34 g/mol | |
Source | PubChem | |
URL | https://pubchem.ncbi.nlm.nih.gov | |
Description | Data deposited in or computed by PubChem | |
Retrosynthesis Analysis
AI-Powered Synthesis Planning: Our tool employs the Template_relevance Pistachio, Template_relevance Bkms_metabolic, Template_relevance Pistachio_ringbreaker, Template_relevance Reaxys, Template_relevance Reaxys_biocatalysis model, leveraging a vast database of chemical reactions to predict feasible synthetic routes.
One-Step Synthesis Focus: Specifically designed for one-step synthesis, it provides concise and direct routes for your target compounds, streamlining the synthesis process.
Accurate Predictions: Utilizing the extensive PISTACHIO, BKMS_METABOLIC, PISTACHIO_RINGBREAKER, REAXYS, REAXYS_BIOCATALYSIS database, our tool offers high-accuracy predictions, reflecting the latest in chemical research and data.
Strategy Settings
Precursor scoring | Relevance Heuristic |
---|---|
Min. plausibility | 0.01 |
Model | Template_relevance |
Template Set | Pistachio/Bkms_metabolic/Pistachio_ringbreaker/Reaxys/Reaxys_biocatalysis |
Top-N result to add to graph | 6 |
Feasible Synthetic Routes
Q1: What is the significance of Tetrakis(2-propynyloxymethyl)methane in material science?
A: Tetrakis(2-propynyloxymethyl)methane (TPOM) is a tetrafunctional molecule containing four alkyne groups. These alkyne groups make it a valuable building block in polymer chemistry, particularly for creating hydrogels through a highly efficient reaction called copper(I)-catalyzed azide–alkyne cycloaddition (CuAAC), also known as "click chemistry" [, , ].
Q2: How is Tetrakis(2-propynyloxymethyl)methane used in hydrogel preparation?
A: TPOM acts as a crosslinking agent in hydrogel synthesis [, , ]. When reacted with polymers possessing azide groups (N3) in the presence of a copper catalyst, TPOM's alkyne groups form stable triazole rings with the azide groups. This crosslinking reaction creates a three-dimensional network that can trap water molecules, resulting in the formation of a hydrogel.
Q3: What are the advantages of using Tetrakis(2-propynyloxymethyl)methane in click chemistry reactions for hydrogel synthesis?
A3: The CuAAC reaction utilizing TPOM offers several advantages for hydrogel preparation:
- High Efficiency: CuAAC is known for its high yields and fast reaction rates, making it an efficient method for crosslinking polymers [, , ].
- Mild Conditions: The reaction proceeds under mild conditions (room temperature, aqueous solutions), minimizing the risk of unwanted side reactions or degradation of sensitive biomolecules [, , ].
- Bioorthogonality: The azide and alkyne groups involved in CuAAC are generally absent in biological systems, making this reaction highly selective and biocompatible, suitable for biomedical applications [, , ].
Q4: Can you provide examples of Tetrakis(2-propynyloxymethyl)methane being used to create hydrogels with specific properties?
A4: Research demonstrates the versatility of TPOM in tailoring hydrogel properties:
- Amphiphilic conetworks: TPOM was used to create amphiphilic conetworks with well-defined structures, exhibiting ordered nanophase separation and tunable swelling properties in both water and organic solvents []. These materials hold potential for controlled drug release applications.
- Interpenetrating Polymer Networks: Researchers have synthesized interpenetrating polymer networks (IPNs) using simultaneous click chemistry with TPOM and atom transfer radical polymerization [, ]. These IPNs demonstrated high swelling ratios, good mechanical properties, and antifouling characteristics, suggesting potential uses as biomaterials.
Disclaimer and Information on In-Vitro Research Products
Please be aware that all articles and product information presented on BenchChem are intended solely for informational purposes. The products available for purchase on BenchChem are specifically designed for in-vitro studies, which are conducted outside of living organisms. In-vitro studies, derived from the Latin term "in glass," involve experiments performed in controlled laboratory settings using cells or tissues. It is important to note that these products are not categorized as medicines or drugs, and they have not received approval from the FDA for the prevention, treatment, or cure of any medical condition, ailment, or disease. We must emphasize that any form of bodily introduction of these products into humans or animals is strictly prohibited by law. It is essential to adhere to these guidelines to ensure compliance with legal and ethical standards in research and experimentation.